Manual on Treatment for Small Water Supply Systems
Manual on treatment for small water supply systems
This manual is adapted from the Manual on Treatment for Small Water Supply systems, final report DETR/DWI 4936/1 (March 2001), produced under contract by WRc. It has been updated by DWI following lessons learnt since the implementation of the Private Water Supplies Regulations 2009/10 (England/Wales).
Original report
Manual on treatment for small water supply systems
Final Report to the Department of the Environment, Transport and the Regions
Report No: DETR/DWI 4936/1
March 2001
Authors: P J Jackson, G R Dillon, T E Irving and G Stanfield
Contract Manager: P J Jackson
Contract No: 12281-0
DETR Reference No: DWI 70/2/137
Contract Duration: May 2000 – March 2001
WRc-NSF Ltd, Henley Road, Medmenham, Marlow, Buckinghamshire SL7
2HD.
Preface
This manual is intended to assist local authorities in the UK in discharging their responsibilities for private water supplies. It may also be of use to anyone, in any country, who may be responsible for, or called on to give advice upon the treatment of private water supplies, in the context of their own national regulations.
Some 50,000 private water supplies serve approximately one percent of the population of England and Wales. In Scotland there are about 20,000 private supplies and there are also significant numbers in Northern Ireland. Wholesomeness standards are prescribed in the 1998 EC Drinking Water Directive. In the UK, regulations incorporate those wholesomeness standards and specify the duties of local authorities in respect of risk assessment, sampling and analysis of private supplies.
Those with responsibilities for assessing, monitoring or improving small water supply systems need to have access to technical advice on a range of issues. These issues include selection and protection of water sources as well as the specification of treatment necessary to improve the quality of water supplies.
This manual provides advice on source selection and protection and on the properties and likely sources of contamination of water. The application of water treatment processes is described, as are a number of small-scale devices that may find application in the treatment of private supplies.
1. Introduction
1.1 Background
Water is essential to sustain life and an adequate supply of good quality drinking water should be available to consumers. International guidelines on drinking water quality are published by the World Health Organization1. Within the European Union drinking water is subject to specific quality standards set out in the EC Directive on the Quality of Water Intended for Human Consumption (98/83/EC2)2, which is known as the Drinking Water Directive and takes into account the WHO guidelines.
The water quality standards laid down in the Drinking Water Directive apply to all public and private water supplies intended for drinking, cooking, food preparation and other domestic purposes.
1 WHO (2011). Guidelines for drinking water quality. Fourth Edition. World Health Organization, Geneva.
2 “Drinking Water Directive”. EC (1998). Council Directive of 3 November 1998 on the quality of water intended for human consumption. Official Journal of the European Communities, No L330, 5th December 1998, pp 32-52 (98/83/EC).
1.2 Private supplies
A private water supply is any water supply that is not provided by a statutory water undertaker. The responsibility for its maintenance and repair lies with the owner or person who uses it. Approximately one percent of the population of England and Wales are served by private water supplies. There are also significant numbers in Scotland and Northern Ireland.
A private water supply can serve a single household or it may serve many properties or commercial or industrial premises. The water source could be a borehole, well, spring, lake, stream or river.
In England, Wales and Scotland local authorities are required to keep themselves informed about the quality of all water supplies in their areas. They are responsible for monitoring private supplies used for drinking, washing, cooking or food production purposes and can require improvements to be carried out where necessary. In Northern Ireland these responsibilities rest with the Drinking Water Inspectorate. District Councils and the Department of Agriculture and Rural Development also have interests in private water supplies in Northern Ireland.
1.3 Construction products and chemicals in contact with drinking water
Chemicals used in water treatment and construction products (e.g. plastic pipes and coatings), used in direct contact with water in water supply systems can adversely affect water quality. Possible effects include the addition of potentially toxic contaminants, which may be present in treatment chemicals, or that may leach from the materials from which pipes and coatings are made. This could also result in aesthetic impacts on the taste and appearance of water, which are noticeable by the consumer.
The Drinking Water Directive requires Member States to ensure that substances and materials (including associated impurities) used in water treatment and distribution do not cause a risk to public health. This is implemented in England through regulation 5(1) and in Wales through regulation 4 of the Private Water Supplies Regulations (2009 and 2010), which only permits the use, by operators of private supplies, of products and substances which will not cause an adverse impact on water quality.
These problems are best addressed through controls on the quality of chemicals and construction products used in water supply systems, from the point of collection to the point-of-use.
In Europe the implementation of schemes to control product quality is currently left to individual Member States. Work is ongoing between UK, Portugal, Netherlands, Germany and France to establish a system of mutual recognition of products and substances approved in one Member State.
A wide range of European standards for drinking water treatment chemicals has been published. Individual Member States, including the UK, operate approval schemes for construction products intended to be used in contact with drinking water. Operators of private water supplies are advised to use treatment chemicals and products that are permitted for use under Regulation 5(1) (Regulation 4 in Wales) of the Private Water Supplies Regulations or appear on the Secretary of State’s List of Approved Products. A published list for private water supplies may be found on the DWI website: (Transitional List of Approved Products)
2. Properties and contaminants of water
2.1 Introduction
The methods used to treat raw water will depend on the properties of the water and the presence and concentrations of any contaminants. Groundwater usually has low levels of colour and turbidity and consistent microbiological quality, although water from shallow wells and some springs may be more variable. Particular problems may include high or low pH and alkalinity and high concentrations of iron, manganese, nitrate, chlorinated solvents or pesticides. Surface water may have high levels of colour and turbidity and exhibit poor microbiological quality. Quality may be variable and deteriorate following periods of heavy rainfall. Other problems may include low pH and alkalinity and high concentrations of aluminium, iron, manganese, nitrate or pesticides.
2.2 Microbiological parameters
Water used as a source for any private supply of drinking water may be of unknown origin and originate from a catchment prone to persistent or intermittent microbiological contamination. This may be derived from an environmental source, such as soil, the faeces of livestock or other domestic animals, wildlife or sanitation systems such as septic tanks. Consequently it is essential that raw water is adequately treated before it is used for domestic purposes (as defined in the Water industry Act 1991). Because no single method of treatment can be expected to remove all types of pathogenic agents, a multiple barrier approach in the form of two or more sequential treatment processes is recommended.
The microbiological quality of drinking water has traditionally been assessed by monitoring indicator bacteria (coliforms, E. coli, and enterococci). Indicator bacteria comprise a range of environmental and enteric bacterial species that are themselves not necessarily pathogenic, but their presence in drinking water is regarded as being indicative of poor quality and/or a potential microbiological health risk. E.coli is a thermotollerant coliform, the presence of which is specifically an indicator of faecal pollution. Similarly, although not in the coliform group, the presence of enterococci in a sample indicates faecal contamination. Although indicator organisms are adequate for monitoring purposes, their absence in a sample cannot completely be relied upon to indicate that the water is compliant with quality standards and free of pathogens at all times. This is especially true of pathogens that are environmentally more robust, or can better survive treatment than the indicators. In these circumstances an indicator may be absent, but low numbers of pathogens could still be present.
An example of such a pathogen is Cryptosporidium. This micro-organism is very much more resistant than faecal indicators, such as E. coli and enterococci, to the destructive action of disinfectants. This is because it has the ability to form spore-like bodies called oocysts that make it less vulnerable to the disinfectant properties of chlorine and similar compounds. If disinfection is the sole treatment process used the numbers of Cryptosporidium oocysts will remain unchanged whereas the numbers of E. coli (and coliforms) may be reduced to undetectable levels.
The use of appropriate filtration (i.e. <1µm) before disinfection is more effective because an appropriate physical treatment barrier will remove oocysts. In addition filtration will remove much of the particulate material present which could otherwise reduce the effectiveness of disinfection by creating an oxidant demand and/or shielding microbes from the effects of the disinfectant. This is a good example of why a multiple rather than a single barrier approach is preferred. In addition the use of multiple barriers will allow some protection to remain even if one process fails.
Despite the possible shortcomings in the use of bacterial indicators as a measure of the microbiological safety of a water supply, monitoring for coliforms and E. coli is still recommended and standards of quality are expressed in terms of these organisms. The reason for this is that monitoring for the pathogens themselves remains rather uncertain because methods of analysis are relatively insensitive and costly compared to those for indicator bacteria. Additionally the absence of one pathogen will not guarantee the absence of others.
Monitoring needs and treatment requirements should however be the subject of regular review and if a need is identified, through for instance, an outbreak due to a specific pathogen or a change noted in the risk assessment for a source, treatment strategies and monitoring requirements may need to be changed.
Growth of algae can be a problem in some surface water sources and in uncovered water storage reservoirs. Algae are naturally present in all inland waters and concentrations can increase and decrease rapidly depending on climatic factors and the availability of essential nutrients. Algae can cause taste and odour in water and some cyanobacteria (usually known as blue-green algae) release toxins that may prejudice the recreational use of water. Although algal toxins have not been identified in drinking water supplies at concentrations that would affect health, it would be prudent to avoid the use of water sources that exhibit high concentrations of such algae.
With the consent of the relevant environmental authorities, it may be possible in some circumstances to adopt measures to minimise the development of excessive algal blooms. The addition of iron salts to precipitate the nutrient phosphate is one measure, although it may not represent a long-term remedy to the problem. In the past, dosing of copper sulphate has been used to reduce growth of algae in reservoirs but its efficacy is questionable. The forced circulation of reservoirs to keep water mixed is another measure. Blooms of algae occur mainly at the surface of reservoirs whereas water is abstracted at depth. Some forms of water treatment, including ozonation, activated carbon adsorption and addition of potassium permanganate can be effective in removing algal toxins. Algae are normally removed as particulate matter during water treatment but some algae can pass through screens and filters or reduce the efficiency of filtration or sedimentation systems.
2.3 Microorganisms associated with outbreaks and private supplies
2.3.1 Review of outbreaks
Being generally smaller, less well equipped than public supplies, and being subject to less stringent surveillance and regulatory requirements private supplies by their very nature are more likely to suffer water quality failures. A review was conducted, on behalf of the Inspectorate, into the number of outbreaks of gastrointestinal infections associated with private water supplies between 1970 and 2009. In the period between 1970 and 2000 there were 25 outbreaks associated with private water supplies. The strength of association between illness and drinking from a private supply was strong in 12 of these outbreaks. In 7 of the identified outbreaks, the strength of association was probable, whilst for the remaining 6 outbreaks the strength of the association was weaker and recorded only as possible. Over half of the identified outbreaks were caused by Campylobacter spp. (13/25, 52%). Other pathogens implicated in outbreaks included Cryptosporidium (4 outbreaks), Streptobacillus moniliformis (1 outbreak), E. coli 0157 (1 outbreak), Giardia (1 outbreak). These included one outbreak of mixed pathogens (Cryptosporidium and Campylobacter). From 2001 to 2009 there were twelve outbreaks. However, only one outbreak had strong evidence of an association with a private supply. For nine of the outbreaks, the strength of evidence for the role of the private supply was probable, and there was one outbreak scored as possible. Identified pathogens involved in the outbreaks included Campylobacter (7 outbreaks), E.coli 0157 (4 outbreaks), Cryptosporidium (3 outbreaks), Salmonella (1 outbreak) and norovirus (1 outbreak). These include 4 outbreaks where more than one outbreak pathogen was detected amongst cases within the outbreak. There were no identified outbreaks caused by unknown pathogens.
As is commonly seen with such private supplies the main pathogens present in these outbreaks would normally be removed by the conventional water treatment systems used for mains drinking water such as chlorination (for the bacteria and viruses) and flocculation, filtration and UV treatment (for these plus the chlorine resistant parasites, including Cryptosporidium). Outbreak investigations typically identified evidence of contamination by livestock, poor conditions surrounding the PWS and inadequate treatment practices. The control measures introduced for implicated supply included interim advice to boil water, chlorination of water and flushing of water distribution systems3.
3 DWI Report WT1269 “Infectious diseases associated with private water supplies in England and Wales from 1970 to 2009”
2.3.2 Campylobacter
The genus Campylobacter is common in the faeces of a wide variety of wild and domesticated animals. In the UK Campylobacters are the main causes of food- related gastroenteritis and although Campylobacter does not multiply readily outside its natural habitat, it can survive in water for some time. This organism is destroyed by disinfection with, for instance, chlorine, ozone or ultraviolet (UV) light and so any organisms passing through the earlier stages of water treatment should not remain viable in the treated water. However, the action of disinfectants can be hindered if the water has not been treated adequately by the removal of dissolved organic material and particulates. The latter can have a shielding effect particularly where UV disinfection is being used.
2.3.3 Cryptosporidium
Cryptosporidia are tiny parasitic animals. Some species cause the diarrhoeal disease cryptosporidiosis in humans. They produce robust spores, called oocysts, which endure for long periods in the environment, including in water. They are excreted by their host animals and therefore faeces of cattle, sheep and other animals, as well as human sewage are the main sources of cryptosporidium contamination in private water supplies.
Physical treatment processes (coagulation, sedimentation and filtration) can provide an effective barrier, preventing the parasite from being present in treated water. Membrane filters with sufficiently small pore sizes will also remove oocysts. Chlorination at the concentrations used in water supply does not inactivate oocysts and a combination of robust and effective catchment control measures, physical barriers and disinfection is required to ensure protection from cryptosporidium.
Private supplies derived from surface waters and from some springs will be vulnerable to contamination with Cryptosporidium, particularly in catchments where livestock farming is common. In these circumstances the use of a suitable filtration stage in treatment is advisable to physically remove Cryptosporidium oocysts as well as particulates, which could be shielding other microorganisms from disinfection. Filtration should be followed by disinfection using chlorine or another suitable and approved disinfectant, to destroy any remaining viruses and/or bacteria.
In reality UV is a more common method of microbiological treatment employed by owners of small private supplies. UV will deactivate Cryptosporidium oocysts but is only effective at when the UV light is of adequate intensity. Further to this the equipment must be operated and maintained according to the instructions for the particular model in use. Since turbidity greater than 1NTU may shield Cryptosporidium oocysts and other microorganisms from disinfection it is advisable to install a suitable filter prior to the UV unit.
2.3.4 Giardia
Giardia is a group of tiny parasitic animals which grow in the intestinal tracts of host animals. It causes a diarrhoeal disease in humans and can be transmitted by direct contact with infected animals and humans, or by consumption of water, food or beverages contaminated by the faeces of infected humans or animals. Giardia forms robust spores called cysts that are infectious and these survive well in water.
The cysts show greater resistance to UV, chlorine, and ozone than bacteria and viruses, but they are less resistant than Cryptosporidium oocysts. Giardia cysts are inactivated by boiling or pasteurisation.
Outbreaks associated with drinking water can occur where human or animal faeces contaminate the supply and the water is inadequately treated. Private supplies should be regarded as being a high microbiological risk if the catchment is prone to contamination by the faeces of animals, if there is a rapid route for recharge to reach the raw water intake and if there are inadequate treatment barriers.
2.3.5 Escherichia coli serotype O157
The bacterium E. coli O157 is a cause of severe disease in Britain. Although most outbreaks and individual illnesses have been due to contaminated food, the organism has been implicated in outbreaks associated with water supplies. In the UK this has been confined to private supplies and there has been a strong association with possible contamination from animal faeces.
Cattle and sheep, and so foods of animal origin, are amongst the most common vehicles of infection from E. coli 0157. Large numbers are excreted in the faeces of infected animals even though the animal may be showing no symptoms of disease. A well maintained multiple barrier treatment strategy including a suitable adequate disinfection stage (chlorination or UV) therefore provide good protection against E. coli 0157.
There are no specific standards for E. coli O157 in drinking water but, as mentioned above, the detection of any E. coli in water should be regarded as evidence that E. coli O157 could be present or that the supply is susceptible to breakthrough by this organism, or indeed other pathogens.
2.3.6 Streptobacillus
Streptobacillus is a bacterium which can be found in private water supplies. It is derived from animals and some types can cause Streptobacillary fever. However, it is a rare infection in the UK.
The small size of this bacterium may allow it to pass through water treatment filters, particularly if there has not been a coagulation stage. However, it is susceptible to disinfectants although it does have the ability to increase its tolerance of disinfection. Thus a well-maintained multiple barrier system would provide the best protection.
2.3.7 Enteric viruses
There are several types of viruses which can be present in faeces and can cause viral enteritis. There have been several relatively large outbreaks (each of more than 100 cases) of viral enteritis associated with private water supplies derived from surface water. In each of these there was evidence of faecal contamination of the supply which had either been inadequately chlorinated, or not disinfected at all. Birds also carry viruses and can contaminate reservoirs and open cisterns.
Although filtration will remove viruses to some degree the primary treatment barrier is disinfection, either by chlorine or UV irradiation. For these to be most effective water must be conditioned by filtration (to remove particulates and aggregates of viruses) before disinfection. Where disinfection is the only treatment being employed, it must be maintained and its performance monitored regularly to optimise disinfectant activity for the prevailing water conditions.
2.3.8 Salmonella paratyphi
Salmonella paratyphi A and B cause the illness paratyphoid fever, which is usually food-borne, although cases of water-borne outbreaks due to Salmonella paratyphi B have been recorded in the UK. Salmonellae would be expected to respond to water treatment processes in a similar way to coliform organisms and E. coli and would have a similar susceptibility to chlorination or other disinfection processes. A well-maintained multiple barrier system would therefore give good protection.
2.4 Chemical contaminants
2.4.1 Aluminium
Aluminium is a natural constituent of many raw waters, particularly acidic surface waters derived from upland catchments. Aluminium can deposit within the distribution system and give rise to aesthetic problems such as cloudiness and discolouration. The UK drinking water quality regulations include a national standard for aluminium of 200µg/l, which is based on avoiding aesthetic issues rather than being health based. Aluminium in raw water can be removed by coagulation and filtration. The use of aluminium sulphate as a coagulant in water treatment should normally result in a residual concentration of no more than 50 to 100µg/l. Aluminium in small water supplies can be removed by use of filtration or membrane techniques.
2.4.2 Ammonia
Most natural waters contain traces of ammonia or ammonium compounds. The ammonia found in water may occur naturally or it may indicate that recent pollution has taken place. Certain anoxic groundwaters may contain elevated concentrations of ammonia (greater than 1mg/l as NH4) resulting from natural ammonification and denitrification processes. Ammonia may also be derived from the decay of organic material in the soil resulting in small concentrations in water. Unpolluted river waters rarely contain more than 0.05mg/l NH4. The presence of ammonia could indicate contamination from human or animal sources and elevated concentrations in water should be investigated to ascertain the cause. The UK drinking water quality regulations set an indicator parameter value for ammonia of 0.5mg/l NH4. However, the appearance of even small amounts of ammonia (e.g. above 0.05mg/l NH4) in a groundwater which is normally free from ammonia warrants further investigations. Ammonia can be removed from water by aeration (after increasing the pH to 11), ion-exchange, biological denitrification and breakpoint chlorination. Of these, only chlorination is likely to be applicable to small supplies. Chlorination converts ammonia to chloramines (see Section 5.9.2) which are less potent disinfectants than chlorine itself and can give rise to taste and odour complaints. Therefore, when designing chlorination systems for ammonia containing waters, the chlorine capacity must be sufficient to produce a free chlorine residual and prevent the formation of taste and odour causing compounds.
2.4.3 Arsenic
Arsenic is introduced into water through the dissolution of minerals and ores, from industrial effluents, and from atmospheric deposition. Concentrations in groundwater in some areas are sometimes elevated as a result of water-rock interaction in the aquifer. The most prevalent species of arsenic in water are inorganic. The predominant form is either arsenic (V) (arsenate), which predominates in oxidising conditions, or arsenic (III) (arsenite), which is present in reducing conditions. Inorganic arsenic is a documented human carcinogen. A relatively high incidence of skin cancer and possibly other cancers that increase with dose and age has been observed in populations ingesting water containing high concentrations of arsenic. The standard for arsenic in the UK is 10µg/l.
Arsenic (V) can be removed effectively by iron or aluminium coagulation. If present as Arsenic (III) then pre-oxidation (e.g. using chlorine) is required. Other potential removal techniques include ferric oxide, activated alumina, ion exchange and reverse osmosis.
2.4.4 Iron and manganese
Iron and manganese derived from minerals and sediments can be present in particulate or dissolved forms in groundwater and surface water. Iron and manganese concentrations in surface water are usually less than 1mg/l but much higher concentrations (up to 50mg/l Fe and 30mg/l Mn) can be encountered in groundwater. Iron can also arise from corrosion of ferrous pipework and chemicals used in treatment processes (coagulation).
Iron and manganese suspensions cause aesthetic problems including metallic taste and discoloration of water fittings and laundry. High dissolved iron and manganese concentrations can also increase chlorine demand and thus reduce the efficiency of chlorine disinfection and can interfere with the efficiency of UV disinfection by coating treatment units. The UK drinking water quality regulations include national standards for iron and manganese of 200µg/l and 50µg/l respectively. Iron and manganese can be removed by filtration although oxidation, coagulation and sedimentation may be required for high concentrations particularly if the metals are in dissolved form. An oxidation (aeration) process is invariably required when groundwater contain more than 1mg/l of dissolved iron or manganese.
2.4.5 Lead
The concentration of lead in raw water rarely exceeds 20µg/l but higher concentrations do occur in water drawn from strata containing galena or other lead ores. High concentrations of lead in drinking water are usually caused by the dissolution of lead (plumbosolvency) from lead pipework, tank linings or historic leaded alloys in water fittings. Traces of lead may also be derived from lead solder and from PVC pipes containing lead-based stabilisers. Lead based plumbing products are no longer approved for use in drinking water systems. The UK drinking water quality regulations specify a standard for lead of 10µg/l. For small water supply systems the best approach to reducing lead concentrations is the replacement of lead containing materials with non-leaded alternatives. However, treatment methods are available to reduce plumbosolvency. Water that has been standing in lead pipes for long periods, for example overnight, should not be drunk. In these circumstances, the tap should be run to clear the pipes before taking water for drinking or cooking.
2.4.6 Nitrate
Nitrate (NO3–) occurs naturally in water as a result of the oxidation of ammonia, which is released during mineralisation of organic nitrogen. In some areas agriculture is the major source of nitrate in surface water and groundwater. The discharge of nitrate containing effluents from sewage treatment works contributes to the concentration of nitrate in some surface water. The UK drinking water quality regulations specify a standard for nitrate of 50mg/l as NO3, although in certain circumstances, where the concentration is less than 100mg/l a private household may not have to take action (see DWI Guidance Note: Nitrate and Private Water Supplies) . In addition the water must satisfy the following formula, where the square brackets signify the concentrations in mg/l of nitrate and nitrite:
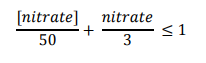
Ion-exchange, biological de-nitrification and certain membrane processes can reduce nitrate concentrations. Of these, only ion-exchange and membrane processes are likely to be practicable for small water supplies. It may be appropriate to consider controls over agricultural activities within catchment areas as a long term means of reducing the leaching of nitrate into water supplies, if these are the source.
2.4.7 Pesticides
The use of pesticides for agricultural and non-agricultural purposes is widespread and in the UK there are approximately 450 different active ingredients in pesticides licensed for use. In surveys of UK surface and groundwater sources, the most commonly reported pesticides are atrazine, simazine and dieldrin. Controls on agricultural uses and withdrawal of approvals for non-agricultural uses of these esticides were introduced in the UK in 1992. Other pesticides commonly reported in water sources used for public supplies include isoproturon, mecoprop and chlorotoluron. Recently, metaldehyde has also emerged as a pesticide of concern due to the inability of conventional water treatment process to remove it.
The UK drinking water quality regulations specify standards of 0.1µg/l for individual pesticides and 0.5µg/l for total pesticides. These standards do not have any toxicological basis. Concentrations of individual pesticides found in typical surface waters can be reduced to less than 0.1µg/l by adsorption on granular activated carbon (GAC) with an empty bed contact time of typically 15 to 30 minutes, depending on the pesticide, its concentration, GAC type and system design. Ozonation will also reduce pesticide concentrations to varying degrees. It is unlikely that treatment to remove pesticides from small supplies will be practicable and where significant concentrations are detected it will be necessary to consider provision of an alternative supply.
2.4.8 Chlorinated solvents
Contamination of groundwater by chlorinated solvents can result from accidental spillage of chemicals, leakage from underground storage tanks, leakage from disposal sites and deliberate discharges to soakaways. The rate of transport of the solvents through the aquifer is dependent on the properties of the aquifer. Rapid transport can occur if the overlying geological material is highly porous or fissured. Contaminated water can travel large distances, making it difficult to pinpoint the source of pollution.
The UK drinking water quality regulations specify standards of 3µg/l for 1,2-dichloroethane and 10µg/l for the sum of the detected concentrations of tetrachloroethene and trichloroethene. Solvent concentrations can be reduced by aeration or activated carbon adsorption. Activated carbon adsorption is likely to be the method of choice for small water supplies although provision of an alternative supply may be an economic consideration.
2.4.9 Disinfection by-products
Trihalomethanes (THMs)
There are a large number of chemical species which may form as a result of the disinfection process and result in taste and odour issues. When chlorine is used as the disinfectant the most common by-products are THMS and so these are of significance to private water supplies.
THMs are formed as a result of reactions between chlorine and some organic substances present in raw water. Highly coloured surface waters which contain humic and fulvic acids are particularly prone to THM formation. The UK drinking water quality regulations specify a standard for Total THMs of 100µg/l for the sum of the detected concentrations of four specified THMs (trichloromethane, dichlorobromomethane, dibromochloromethane and tribromomethane).
THM formation can be controlled by reduction of the organic content of the water before disinfection, modification of the disinfection practice or the use of disinfectants other than chlorine, such as ultraviolet radiation or ozone. However, ozone may cause other problems by reacting with organic matter. THMs precursors can be removed to some extent by adsorption on activated carbon.
Bromate
Bromate is not present in source waters but is formed by oxidation of bromide if ozonation is used for water treatment. Elevated bromide concentrations occur where saline intrusion occurs into fresh aquifers in coastal areas, increasing the potential for bromate formation during ozonation. Bromate is also a potential impurity in sodium hypochlorite, a commonly-used disinfectant. The UK drinking water quality regulations specify a standard for bromate of 10µg/l.
The formation of bromate during ozonation depends on several factors including concentrations of bromide, ozone and the pH. It is not practicable to remove bromide from the raw water and it is difficult to remove bromate once formed, although granular activated carbon filtration has been reported to be effective under certain circumstances. Bromate formation can be minimised by using lower ozone dose, shorter contact time and a lower residual ozone concentration.
2.5 Physical and chemical properties of water
2.5.1 pH value
The pH value of water is a measure of its acidity or alkalinity. Pure water is very slightly ionised into positively charged hydrogen ions (H+) and negatively charged hydroxide ions (OH–). Water is neutral when the numbers of hydrogen ions and hydroxide ions are equal. When the concentration of hydrogen ions exceeds that of hydroxide ions, the water is acidic and has a pH value less than 7. Conversely, when the concentration of hydroxide ions exceeds that of hydrogen ions, the water is alkaline and has a pH value greater than 7. The pH scale is logarithmic, therefore a change in pH value of one unit represents a tenfold change in the concentrations of hydrogen or hydroxide ions.
Acidity in raw water can result from the dissolution of carbon dioxide to produce weak carbonic acid. Groundwater and surface water may also contain organic acids produced during the decomposition of vegetation. Surface water derived from a peaty moorland catchment may have a pH value as low as 4. Alkaline waters result almost entirely from the dissolution of the bicarbonate, carbonate and hydroxide salts of calcium, magnesium, sodium and potassium, for instance from limestone aquifers. Soft acidic waters can cause corrosion of pipework and the dissolution of metals such as copper, zinc and lead. Hard alkaline waters can cause scale formation and some hard waters may also be plumbosolvent.
The UK drinking water quality regulations include pH as an indicator parameter and specify a minimum pH of 6.5 and a maximum pH of 9.0. In water treatment, the pH value can be changed by aeration and alkali or acid dosing.
2.5.1 Hardness
Water hardness is caused by dissolved salts of calcium and magnesium. Total hardness consists of temporary and permanent hardness. Temporary hardness is caused almost entirely by the carbonates and bicarbonates of calcium and magnesium. Temporary hardness is precipitated by evaporation and boiling. Permanent hardness is caused almost entirely by the sulphates and chlorides of calcium and magnesium. Permanent hardness is not precipitated by boiling.
The hardness of waters, expressed in mg/l CaCO3 (calcium carbonate), can be classified as shown below:
Table 1: Water Hardness
Water | Hardness (mg/l CaCO3) |
---|---|
soft | up to 50 |
Moderately soft | 50 – 100 |
Slightly hard | 100 – 150 |
Moderately hard | 150 – 200 |
Hard | 200 – 300 |
Very hard | over 300 |
The Drinking Water Directive and the UK drinking water quality regulations do not specify standards for hardness, calcium or magnesium. Softening can be achieved by lime-soda softening, where the addition of lime (Ca(OH)2) and sodium carbonate (Na2CO3) to the water causes the hardness compounds to precipitate. An alternative method, common in domestic water softeners, is ion-exchange (base exchange), whereby the calcium and magnesium ions in the water are replaced by sodium ions. Where water is softened by base exchange softening it is important to provide an unsoftened outlet for potable purposes. Installation of a softener just before the hot water tank or boiler is a more economical method for preventing precipitation of hardness salts (limescale) than softening the whole supply.
2.5.2 Colour
Water can be coloured by humic and fulvic materials leaching from peat or other decaying vegetation and by naturally occurring salts of iron or manganese. Surface water derived from peaty moorland catchments may be strongly coloured. The characteristic brown colour of such water is variable and often shows a strong seasonal effect, with concentrations being greatest in late autumn and winter. Water derived from lowland rivers can similarly show a seasonal increase in colour following autumn leaf fall.
Water may appear coloured because of material in suspension and true colour can only be determined after filtration. Colour is expressed in mg/l on the platinum-cobalt (Pt-Co) scale, which is equivalent to measurements expressed in Hazen units (°H). The removal of colour from water is necessary not only for aesthetic reasons but also because chlorination of highly coloured waters can give rise to high concentrations of trihalomethanes. High colour also reduces the efficiency of disinfection by UV irradiation, chlorination and ozonation and will also cause fouling of reverse osmosis membranes.
The Drinking Water Directive includes colour as an indicator parameter without a numeric standard but with the requirement “Acceptable to consumers and no abnormal change”. The UK water quality regulations specify a standard of 20mg/l PtCo. Filtration techniques may be applied to small supplies but the efficiency of colour removal is usually relatively poor.
2.5.3 Turbidity
Turbidity is caused principally by inorganic matter in suspension including mineral sediments (e.g. from chalk) and oxides of iron or manganese but organic matter including algae can also cause significant turbidity. Most surface waters show particularly high turbidity following periods of heavy rainfall, whilst groundwater generally shows low to very low turbidity. However, variations following heavy rainfall, for example, may indicate rapid recharge bringing in contaminants from the surface.
Turbidity measurement gives a quantitative indication of the clarity of water and analysis is carried out using a nephelometer. Nephelometers measure the intensity of light scattered in one particular direction, usually perpendicular to the incident light and are relatively unaffected by dissolved colour. Nephelometers are calibrated against turbidity standards prepared from a suspension of formazin. The standard unit of turbidity is the nephelometric turbidity unit or NTU.
Turbidity is removed because high turbidity can impair the efficiency of disinfection and for aesthetic reasons. The UK water quality regulations specify a standard of 4NTU at consumers’ taps with an indicator parameter value of 1NTU in water leaving a treatment works.
Rapid sand filtration or microstraining can remove coarse turbidity and some species of algae. Fine turbidity and many species of algae that may penetrate rapid filters can be removed by slow sand filtration or by coagulation followed by sedimentation or flotation and filtration. A variety of filtration techniques can be successfully applied to small supplies, however, cartridge filters are the most widely employed.
2.5.4 Taste and odour
Sources of taste and odour in source water include decaying vegetation, algae, moulds and actinomycetes. Taste and odour are usually associated with the presence of specific organic compounds released by the source agent which give rise to “earthy” or “musty” taste or odour. Chlorine and the by-products of chlorination can also cause complaints of taste or odour. Relatively high concentrations of iron, manganese and some other metals can impart an unpleasant metallic taste. Domestic plumbing materials and arrangements and in some circumstances water mains may also impart a noticeable taste or odour.
The Drinking Water Directive includes taste and odour as indicator parameters without numeric standards but with the requirement “Acceptable to consumers and no abnormal change”. The intensity of odour and taste is expressed as a Dilution Number, which is the dilution of the sample with odour or taste free water at which the odour or taste is undetectable. The UK water quality regulations specify standards for both odour and taste of 3 dilution number at 25ºC.
Taste and odour are removed principally for aesthetic reasons. Taste and odour can be reduced or removed by aeration, ozonation or adsorption on activated carbon or, where chlorination is the source of taste or odour, by control of the disinfection process.
2.6 Radioactivity
All environmental water contains traces of naturally occurring radionuclides, the concentrations of which depend on the origin of the water. The natural radionuclides of most relevance to drinking water supplies are radon (Rn) and uranium (U). Radon is volatile and as a result it can be released from water as a gas. This is of concern if the release occurs within a confined space with insufficient ventilation.
Radon and uranium are only found in significant concentrations in groundwater in certain parts of the UK, depending on the type of geology from which the groundwater originates. Further advice is available from local authorities, the British Geological Survey and the Radiological Protection Services of Public Health England). These substances are not significant for any surface water sources in the UK.
The concentration of radioactive elements in water is expressed in terms of their activity, in Bequerels per litre (Bq/l). There is a European Commission Directive (2013/51/Euratom) which specifies a parametric value for radon in drinking water supplies of 100Bq/l and an action level of 1,000Bq/l, above which action should be taken to protect public health. The requirements of this Directive have been transposed into the national drinking water quality regulations. There is no official recommended level for uranium and The World Health Organisation recommends a provisional guideline value for total Uranium of 30µg/l. However, there are separate guidance levels for individual uranium isotopes.
Treatment for radon cannot include point-of-use systems fitted to the tap because, being volatile, it is released into the atmosphere whenever water is used. Under-sink treatment using an activated carbon filter is also inadvisable because the filter would become radioactive. Radon removal treatment therefore has to be installed before entry of water into a building and aeration is the preferred treatment technique although other methods are feasible. Uranium removal is best achieved by point-of-use systems (see Section 7).
3. Source selection, protection and monitoring
3.1 Introduction
Water which flows in streams and rivers or which is contained in lakes is called surface water whilst water which percolates into the ground and reaches the water table is called groundwater. The general features of geological formations associated with surface water and groundwater sources are shown in Figure 1.
Figure 1: Groundwater and surface water sources
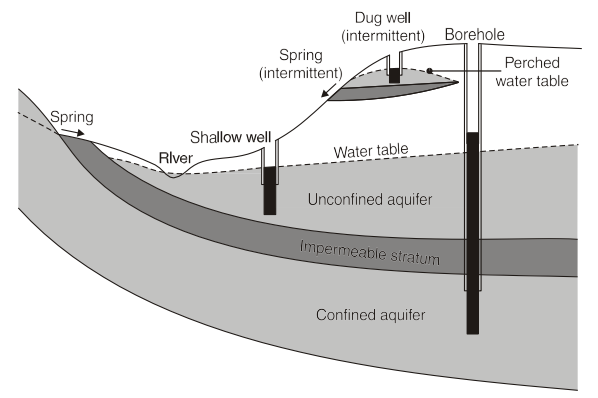
3.2 The hydrological cycle
Precipitation introduces water into the terrestrial environment where it may percolate into the ground, run-off as rivers and streams, or be returned to the atmosphere through evapotranspiration. Precipitation falling on to a soil will wet the soil surface and then infiltrate below ground level where it adheres to the soil particles by a combination of surface tension and molecular attraction to form pellicular water. Such pellicular water escapes the forces of gravity as the attractive forces of surface tension and molecular attraction are such that only evapotranspiration can remove this bound water. Thus precipitation will only penetrate deeper once the soil reaches its field capacity, at which the force of gravity exceeds the attractive forces binding water to soil particles and allows the water to drain downwards. In this region of the soil the voids present are not completely filled with water and so this region of the subsurface is known as the unsaturated zone. As gravity pulls the water down to greater depths the voids become completely filled with liquid and this is termed the saturated zone. Water in this saturated zone is termed groundwater and the boundary between the unsaturated and saturated zone is termed the water table. This separation is not clear-cut and the transition phase between the unsaturated and saturated zone is called the capillary zone or capillary fringe.
If these zones are viewed in terms of pressure then it is found that the unsaturated and saturated zones have different characteristics. The pressure gradient in the unsaturated zone is less than atmospheric pressure, i.e. atmospheric minus capillary pressure, whereas in the saturated zone the voids are completely filled with water at a pressure above atmospheric pressure. Thus the water table may be defined in terms of pressure as the level in the subsurface where atmospheric pressure occurs. These pressure differentials mean that if a well or borehole is excavated into the saturated zone, water will flow from the ground into the well. Water will then rise to a level in the well where the pressures equilibrate. Groundwater can be broadly defined as that water located below the water table, i.e. in the geomatrix (soil, rock or other geological material) where the void area, the space between the constituents of the geomatrix, is approximately 100% occupied by water. These voids or pores can be used to classify groundwater- bearing rocks into two broadly exclusive groups
- reservoirs: geomatrix containing voids that allows liquid to penetrate into the main body of the material;
- non-reservoirs: geomatrix lacking any void space and therefore unable to harbour any liquid.
Reservoirs vary in the degree to which stored water will be released as some may not easily release their stored water e.g. clays are reservoirs but do not release their stored water. This feature of reservoirs requires a further division into permeable and impermeable reservoirs.
Another feature is that groundwater is dynamic, being constantly in motion through the geomatrix. The ease with which water can pass through particular rock strata depends on a combination of the size of the pores and the degree to which they are interconnected resulting from the degree to which the rock is permeable. An aquifer is any rock which contains interconnected pores or fissures which can hold and transfer water and may be defined as a water-bearing rock formation that contains water in sufficient amount to be exploited and brought to the surface by wells.
Geomatrix materials that can serve as aquifers include gravel, sand and sandstone, alluvium, cavernous limestone, vesicular basalt and jointed slate. The different components that combine to produce an aquifer system are shown in Figure 2. It is apparent that there are two distinct types of aquifers: confined or unconfined. In an unconfined aquifer the water table is unrestricted and can thus move up or down through the geomatrix. By contrast a confined aquifer is restrained by an upper layer of impermeable rock, termed an aquiclude, which prevents water moving upwards. As discussed above, the pressure in a confined aquifer will be above atmospheric pressure and this pressure difference will cause water to rise in a well shaft that penetrates the aquiclude. Such wells are termed artesian wells.
To complete the hydrological cycle within the groundwater area, all freshwater found underground must have a source of recharge such as rainfall or leakage from surface drainage such as rivers, lakes or canals. It should be borne in mind that groundwater systems are dynamic with water continuously moving from areas of recharge to areas of discharge with transit times of many years.
Figure 2: Confined and unconfined aquifers
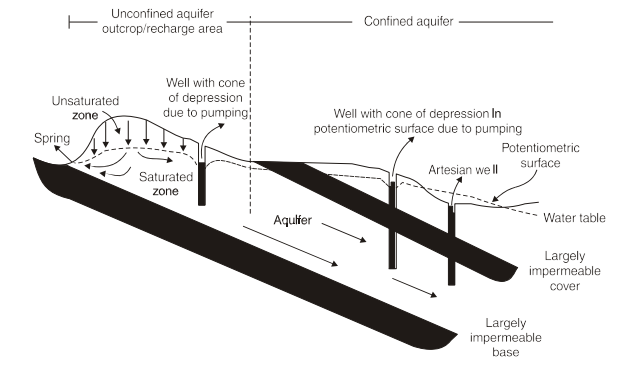
3.3 Source selection
The principal sources of private supplies are springs, wells and boreholes. Streams and rivers are also used but to a lesser extent because of the reduced spatial accessibility of surface water compared to groundwater as well as the more variable quality of surface water. Other, sources of water used in the domestic setting may include rain water (which should be treated as surface water) and sea water (from desalination). Whatever the source, it must consistently yield a quantity of water sufficient to satisfy the requirements of the user.
3.3.1 Streams and rivers
Streams and rivers may be susceptible to pollution and may exhibit variable quality. The risk and extent of pollution depends on the catchment and the activities being undertaken on it. Water derived from upland catchments that are not unduly peaty and not used for agricultural purposes are usually of good chemical quality. However, soft acidic upland waters derived from peaty moorland catchments may be corrosive (to plumbing fittings) and contain relatively high concentrations of dissolved metals. Small streams often exhibit variable quality because of the activities of man and animals within the catchment.
Lowland surface waters are likely to be of poorer quality. The quality of surface waters may show a strong seasonal variability. Colour may be highest in late autumn and winter. Turbidity may be highest following periods of heavy rainfall.
Because of these potential problems, a surface water source is normally only considered for use as a drinking water supply where a groundwater source is unavailable. Water treatment will require a minimum of filtration and disinfection and should be designed for the worst expected raw water quality. A small reservoir or tank installed at the source can provide a period of settlement and reduce the variability in water quality. This tank will require regular inspection and cleaning. Figure 3 shows the construction of brick or concrete reservoirs; pre-cast concrete reservoirs can also be used.
3.3.2 Springs
Th.3.1e quantity of water available from a spring depends on its source. Most reliable are springs issuing from deep aquifers whereas those from perched water tables or supported by flow from fissured limestone or granite aquifers may dry up after a short period without rain. Spring sources can be of good chemical and microbiological quality although springs from shallow or fractured strata may be of variable quality because of surface contamination. The treatment of spring waters is usually simpler than for surface waters because spring water is likely to contain less suspended matter.
Some “spring sources” used for small water supplies are in fact artificial land drains. If the whole drainage system is properly maintained, the quantity and quality of water may be acceptable for a drinking water supply but for assessing treatment needs, a land drain should be regarded effectively as a surface water source. If maintenance is poor, the water quality and flow may decrease. The probability of agricultural pollution must be considered carefully.
Figure 3: Brick or concrete reservoirs

3.3.3 Wells and boreholes
Many small drinking water supplies are derived from wells and boreholes. Wells are usually of large diameter and dug by hand or, more rarely, by a mechanical excavator. Boreholes are of smaller diameter, variable depth and are drilled by a specialist contractor using a mechanical drilling rig.
The quantity of water available will depend on the characteristics of the aquifer and the well or borehole drilled into it, and can be determined by test pumping after construction. A well or borehole that penetrates an extensive aquifer will be the most reliable, where as a well or borehole sunk into a perched aquifer may dry up after a short period without rain.
Water abstracted from deep wells and boreholes may have originated from catchments several miles away. If the aquifer is a porous stratum, such as sand or gravel, the water will have undergone thorough filtration. Such water will usually be of very good quality. Some aquifers, such as limestone or granite strata, may be fissured and the filtration of the water will not have been so thorough. Groundwater abstracted from shallow wells and boreholes may be prone to local pollution unless adequate precautions are taken. However, groundwater is usually of good quality and treatment may consist of disinfection only. However, some groundwater contains high concentrations of iron and manganese, which are usually removed by oxidation and filtration. Others may be polluted by nitrate or pesticides derived from agricultural or other activities or by chemicals from industrial sites.
3.4 Source protection
3.4.1 Streams and rivers
Pollution and natural variations in water quality are the main problems associated with stream and river sources that need to be considered when siting and constructing an intake. Water may be pumped directly from the stream or river or it may be collected from the ground in the immediate vicinity of the stream or riverbank. The advantage of the latter is that where the strata have suitable transmissive properties, supplies taken in this way are naturally filtered and of better quality than the river water itself.
The intake should be located away from any features that might create turbulence during periods of heavy rainfall and increase the turbidity of the water. This means that intakes should not be situated on bends in the stream or river or at places where sudden changes in level occur. Most commonly, intake pipes are situated in the stream or river protected by a strainer to prevent the ingress of debris, fish and vermin. The inlet pipe feeds a settlement tank that allows particulate material to settle. The outlet of the tank, fitted with a strainer, should be situated above the floor of the tank to prevent contamination by sediment. The tank must be built of a material that will not impair water quality and designed to prevent entry of vermin and debris. An example of a slightly more sophisticated intake is shown in Figure 4. The inlet pipe is situated in a small gravel filled tank buried upside down in the stream or riverbank (alternatively, the tank may be buried in the stream or river bed). The water enters the tank through a substantial thickness of riverbank material. This type of infiltration gallery will only be appropriate where the riverbank is sufficiently permeable to allow water to enter the tank at an adequate rate. The intake may suffer a gradual loss of capacity through siltation.
Figure 4: River Source
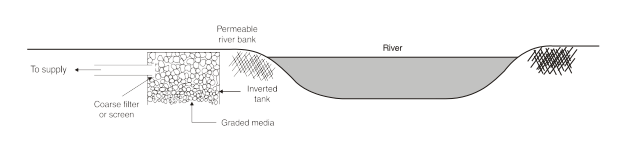
3.4.2 Springs
Spring water can be of good quality but it must be protected from possible contamination once it has reached ground level. In particular, it is necessary to consider the possibility of pollution from septic tanks or from agricultural activities. A small chamber built over the spring, for example as shown in Figures 5 and 6, will protect it from pollution, provide storage for periods of high demand and serve as a header tank. The collection chamber should be built so that the water enters through the base or the side. The top of the chamber must be above ground level and it should be fitted with a lockable watertight access cover. An overflow must be provided and be appropriately sized to take the maximum flow of water from the spring. The outlet pipe should be fitted with a strainer and be situated above the floor of the chamber. The chamber should be built of a material that will not impair water quality and be designed to prevent the entry of vermin and debris. The area of land in the immediate vicinity of the chamber should be fenced off and a small ditch dug upslope of the chamber to intercept surface run-off.
Figure 5: Spring source schematic
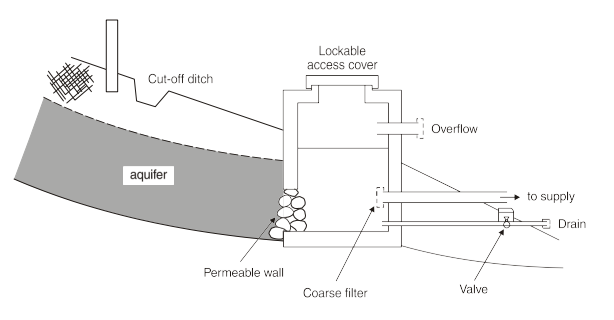
Figure 6: Spring collection chamber

3.4.3 Wells and boreholes
Shallow wells and boreholes are more at risk from contamination than deep wells and boreholes but if built and sited correctly, both may provide good quality water. Similar measures may be taken to protect both sources and an example is shown in Figure 7.
The upper section of the shaft must be lined and sealed against the surrounding material to exclude surface water ingress and, in the case of shallow wells and boreholes, water from the upper layer of the aquifer. Such sanitary seals range in depth depending on the aquifer but all must extend above ground level. Wells are often lined with masonry or concrete pipes and boreholes with steel, plastic or glassreinforced plastic casings and sealed into the ground by a cement grout injected into the annular space between the casing and the surrounding ground. The shaft lining material should not affect water quality.
Where boreholes are drilled through a perched aquifer into lower water bearing strata or several different aquifers, highly variable water quality may be obtained. Use of such boreholes as sources of potable water should be avoided unless the area through the perched aquifer is sealed.
Slotted or perforated borehole linings lining may extend some depth into the aquifer if the bottom section requires support. A gravel packing may be necessary if the borehole penetrates unconsolidated aquifer material to prevent fines from being drawn into the borehole during pumping. At ground level, the well or borehole should be covered by a watertight chamber with a lockable cover. A concrete apron should slope away from the chamber to drain surface water. The well or borehole should preferentially be sited up-hill of, and at least 50m away from, potential sources of pollution which include septic tanks, sewer pipes, cess pools and manure heaps. Typical arrangements for wells and boreholes are shown in Figures 8 to 10.
Figure 7: Well or borehole source schematic

Figure 8: Typical well and submersible pump installation

Figure 9: Typical well and surface pump installation

Figure 10: Borehole headworks

4. Assessment of pollution risk to small water supplies
4.1 Raw water sources
4.1.1 Influence of microorganisms on groundwater quality
The major influence of microorganisms on groundwater quality is in terms of their persistence and mobility in the subsurface environment. Bacteria derived from manmade sources are usually eliminated in the subsurface environment through a combination of physicochemical and biological processes. However, elimination rates are specific for each particular microbial species. Figure 11 illustrates the elimination rates of various bacteria and viruses. Virus survival and migration appear to be controlled by the climate, clay content and moisture-holding capacity of the geomatrix and the specific class of virus involved.
Figure 11: Elimination rates of bacteria and viruses
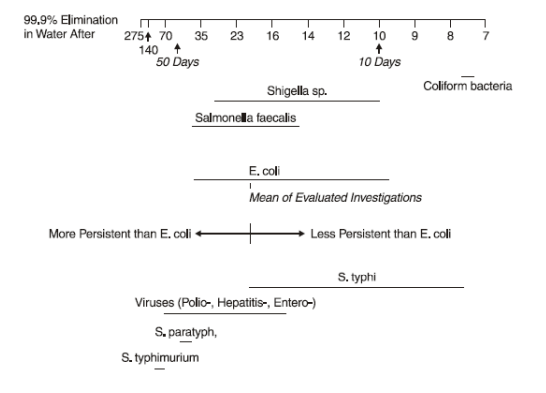
4.1.2 Raw water catchment
Abstraction of water from a well or borehole in an unconfined aquifer lowers the level of the water in the immediate vicinity of the abstraction point. This lowering of the water level is termed drawdown and assuming that the aquifer is homogeneous and no impermeable strata are present it will occur in a broadly circular pattern in plan view. The area of this circle is related to the hydraulic characteristics of the aquifer and the rates of abstraction and recharge. The cross-sectional shape of the drawdown is like an inverted cone with the apex being at or near the base of the well or borehole. This phenomenon is known as the cone of depression. Around each groundwater source an area is created from which water will drain towards the abstraction point. At the outermost edge of this area there will be a groundwater divide, beyond which water will bypass the source and not be drawn towards it. This divide in the flow of groundwater delineates the capture zone for the source.
It is important to distinguish between the capture zone and the cone of depression around the source. The capture zone consists of the up-gradient and down-gradient areas that will drain into the source. In the unlikely event that the water table was perfectly flat, the capture zone would be circular and would correspond to the cone of depression. However, the more common case is for the water table to be sloping due to regional groundwater gradients. In these circumstances the cone of depression and the capture zone will not correspond. This situation arises due to the hydraulic gradient of the water table downstream being dramatically changed through the action of pumping. Water will only flow towards the well where the pumping induced change in the gradient is sufficient to fully reverse the regional groundwater gradient. As pumping continues the area of the capture zone increases and this process will continue until the rate of abstraction is equalled by the recharge over the entire capture zone area. Thus the entire capture zone is known as the source catchment.
Groundwater fed springs may dry up as the water table falls during periods of low precipitation, or they may flow continuously as perennial springs with only slight variations in discharge rate throughout the year. When considering source protection the degree of protection afforded to a spring must be derived from the total spring discharge as the movement of the water through an aquifer system and issuing as a spring at any particular time is unknown. For this reason, protection zones around springs that are used as sources of supply must be derived on the basis of the total flows issuing through the spring.
4.1.3 Protection zone delineation for groundwater sources
Once the source catchment has been identified source protection zones can be delineated. These zones are required to understand the risk of contamination of groundwater being abstracted by considering how quickly potential contaminants in a given zone may reach the source. These source protection zones utilise a tripartite zonation system where the source catchments are subdivided into three roughly concentric zones. Two of these zones are determined by time of travel of the pollutant and the third by the source catchment itself. It should be noted that the shape of the zones is dictated by the characteristics of the aquifer and so in fractured rocks the zones may be elongated and stretch some distance from the source. The source protection zones are as follows4:
4 Environment Agency, ‘Groundwater Source Protection Zones’ from http://apps.environmentagency.gov.uk/wiyby/37833.aspx
Inner zone (Zone 1) – Defined as the 50 day travel time from any point below the water table to the source. This zone has a minimum radius of 50 meters.
Outer zone (Zone 2) – Defined by a 400 day travel time from a point below the water table. The previous methodology gave an option to define SPZ2 as the minimum recharge area required to support 25% of the protected yield. This option is no longer available in defining new SPZs and instead this zone has a minimum radius of 250 or 500 meters around the source.
Total catchment (Zone 3) – Defined as the area around a source within which all groundwater recharge is presumed to be discharged at the source. In confined aquifers, the source catchment may be displaced some distance from the source. For heavily exploited aquifers, the final Source Catchment Protection Zone can be defined as the whole aquifer recharge area where the ratio of groundwater abstraction to aquifer recharge (average recharge multiplied by outcrop area) is >0.75. There is still the need to define individual source protection areas to assist operators in catchment management.
Special interest (Zone 4) – A fourth zone SPZ4 or ‘Zone of Special Interest’ was previously defined for some sources. SPZ4 usually represented a surface water catchment which drains into the aquifer feeding the groundwater supply (i.e. catchment draining to a disappearing stream). In the future this zone will be incorporated into one of the other zones, SPZ 1, 2 or 3, whichever is appropriate in the particular case, or become a safeguard zone.
4.2 Assessment of groundwater vulnerability – soil and land use factors
4.2.1 Vulnerability and risk considerations
The susceptibility of groundwater to microbiological contamination from surface or near-surface derived pollutants is greater under certain conditions than others. At any specific site, groundwater contamination depends on the natural or manmade characteristics of the site. Thus the ease with which the potential pollutant can migrate to the underlying water table or spring source depends on the physical, chemical and biological properties of the local strata. The factors that determine the vulnerability of groundwater to given pollutants or activities are as follows:
- characteristics of the overlying soil
- characteristics of the drift deposits
- nature of the solid geological strata within the unsaturated zone
- depth to groundwater
- nature of the contaminant
Land use is a critical factor and manmade structures or excavations can modify the impact of the factors listed above. Groundwater vulnerability classification is determined by the nature and quantity of material in the unsaturated zone, i.e. above the water table. In the absence of major fissures or cracks within this zone water movement is essentially slow, being confined to interconnected soil pores within an aerobic environment. However, the rate of this movement depends on the moisture content of the soil and will vary throughout the year. The overlying soil provides the potential for interception, adsorption and elimination of bacteria and viruses. Where vertical fissures occur or shattered rock comes close to the earth’s surface, there is the potential for rapid flow of microorganisms to groundwater. These features reduce the ability of the soil and substrate to act as a barrier or filter.
4.2.2 Use of groundwater vulnerability maps
A methodology for classifying soils into three leaching potential classes has been developed by the Soil Survey and Land Research Centre for use in groundwater vulnerability maps. This classification also embraces all Soil Series that have been mapped to-date within the UK, with each soil series being assigned a value corresponding to the ease with which a representative pollutant could move through the soil. The mapping developed from this data may be of use when considering the potential water quality risks for a specific source.
4.2.3 Other factors that influence groundwater vulnerability
A series of site specific factors can contribute towards possible groundwater contamination but in most instances it is not possible to quantify the degree of risk. Examples of these are listed below.
- Physical disturbance of aquifers and groundwater flow. These activities lead to the disturbance of the physical barrier offered by the soil and may provide preferential pathways of water (and contaminant) movement to shallow groundwater. These include most forms of groundwater extraction, landfill operations, nearby borehole construction, any activity which interconnects naturally separate aquifers, existing or modified field drainage schemes that intercept recharge water, quarrying and gravel extraction both above and below the water table.
- Waste disposal to land. Many waste disposal practices have the potential to cause groundwater contamination. In this respect, the Environment Agency has laid down certain regulations, many of them statutory, to ensure specific objectives. For example, there will normally be objections at the planning stage to waste disposal activities that extend to or below the water table within prescribed limits of a source. However, the disposal of slurries and other wastes on agricultural land in the vicinity of a private water supply is not subject to the same regulation, although codes of good agricultural practice do exist.
- Contaminated land, being land currently or previously used in connection with specific potentially polluting activities, such as sewage treatment works, landfill sites and other waste disposal and recycling activities and waste lagoons. Also the Environment Agency will seek to protect water supplies where any of the above activities are to be found in close proximity to a water source.
- The application of liquid effluents, sludge and slurries to land. Three categories of waste are recognised, being controlled wastes (industrial effluent and sludge, both organic and inorganic in nature), sewage sludge and agriculture waste. Where the Environment Agency considers that any of these will give rise to a significant risk of polluting groundwater or surface water, there will be a presumption against spreading or compliance with existing environmental legislation. Farmers should have a waste management plan, with information relevant to suitable land available for spreading liquid effluents, sludge and slurries.
- Discharges to underground strata. Three areas of concern have been identified:
- sewage effluent discharges including septic tank and sewage treatment plant effluents from individual properties or small housing estates
- trade effluent discharges
- surface water discharges that include contaminated runoff from roofs and impermeable areas such as roads, car parks, storage areas and so on.
- Diffuse pollution of groundwater. Diffuse pollution refers to pollution spread over time and space and caused by mechanisms other than local and specific discharges or events. Such pollutants are usually at much lower levels than other sources and are therefore at lower concentrations in the soil water.However, the build-up over a long period can generate potential problems. Diffuse pollution varies in character between urban and rural areas. Within the former, the two most notable examples of pollution arise from industrial sites and discharges from sewage systems. In contrast, within rural areas, the pollutant is not from an individual point discharge but arises from activities connected with intensive arable and livestock farming.
- Additional activities or developments which pose a threat to groundwater quality include miscellaneous activities such as storage of farm wastes and intensive livestock housing, graveyards and animal burial sites, sewage works and storm overflows.
4.2.4 Soil and land use factors underpinning the assessment of surface water vulnerability
In some instances, small water supplies are fed by surface waters. The role of soils in offering protection to these sources is much more limited than that described above for groundwater but nonetheless, differences in soil type will have an influence on the risk of microbiological contamination of these waters. Clearly, the soil has no role where the contaminant is deposited directly into the water body, but where a potential contaminant is deposited near to a water body there are a number of factors which affect the risk of contamination. The main factors are surface runoff which washes the contaminant into the water body and stream extension (both laterally and upslope) which entrains the contaminant.
The degree of surface flow is dependent on the intensity and duration of rainfall, the soil type, slope and land use. In general terms, high intensity rainfall, like that associated with thunderstorms, is likely to initiate overland flow in most soils as the infiltration rate of the soil is exceeded by the rainfall intensity. Low intensity rainfall over a prolonged period of time can also lead to overland flow. In both cases the soil type can have a major influence on the amount of rainfall that can be absorbed before the initiation of runoff. Soils with open, porous structures and with no slowly permeable layer will be able to absorb more than shallow soils or those with slowly permeable layers near to the soil surface. Land use can act both as an interceptor for rainfall (for example, a forest), reducing the amount that reaches the soil surface as well as providing the opportunity for the presence of potential contaminants (for example, open moorland that is grazed by domestic and wild animals).
Stream extension is the process whereby the apparent stream network as seen under dry conditions extends during rainfall, with the development of ephemeral streams and rivulets that occupy topographic hollows and are interconnected with the normal stream network. In many catchments, these streams and rivulets become dry soon after the rain has stopped. However, during rainfall the water flowing along these pathways will often be sufficiently fast and deep to entrain contaminants such as animal faeces. The occurrence of these pathways is difficult to predict from soil maps but some soil types will be more likely to behave in this way than others. During periods of rainfall, the levels of streams and rivers generally rise and may extend out beyond their normal channel to occupy their floodplain. In many small catchments, these floodplains may be only a few metres wide, but any faecal material or other potential contaminants on the surface may be entrained in the stream.
5. Water treatment processes
5.1 Introduction
Larger water supplies serving many properties or commercial or industrial premises usually have shared upstream treatment systems similar in principle to those used at municipal water treatment works. This means that water is fully treated before being supplied to a distribution system from where it will go on to feed consumers. Smaller supplies, such as those feeding individual properties, would normally still be expected to have a suitable form of treatment situated such that it will provide water for drinking and other domestic uses at all points in the property, although there are exceptions which are discussed later (see section 6)
It is essential that the design of any treatment process is based on a full investigation of site conditions, including chemical and microbiological analysis of the water to be treated, a risk assessment and the results of laboratory or pilot scale tests to determine the effectiveness of the process and the chemical dosing requirements. This chapter provides an overview of the basic principles of water treatment; anyone planning to install or upgrade a water treatment process should seek expert guidance.
Where water is used for any domestic purposes it must be wholesome. Requirement for treatment may vary according to rainfall, catchment activity or other reasons.
Although monitoring may indicate that the water is bacteriologically safe some of the time, it is extremely likely that there will be a bacteriological challenge at other times. In practice this means that many supplies will require a disinfection stage unless the supply can be shown by risk assessment and frequent surveillance to be likely to be consistently pathogen free.
It is important to choose equipment, suppliers and consultants carefully. DWI has published a list of products and substances which are approved for use which is available at dwi.gov.uk. This provides information on permitted products, their suppliers and lists some common types of products. Purchasers of water treatment plant and supplies should:
- ensure that potential suppliers are aware of the size and nature of the water supply
- confirm that potential suppliers can supply and install (if required) equipment suitable and approved for use with private water supplies
- establish whether suppliers can provide references relating to similar projects
- if practicable inspect other, similar, installations
- ensure that instructions for use and ongoing management and maintenance requirements of the equipment are provided
The DWI has produced a checklist for treatment which may be found at dwi.gov.uk. This shows basic treatment capabilities of commonly found treatment options.
A range of water treatment processes is covered here and in the following chapter, which deals with point-of-use systems for treatment of water at a single tap. For some contaminants, potentially several techniques could be appropriate. For example, membrane processes can remove a broad spectrum of contaminants but cheaper and simpler alternatives may be just as effective in particular cases. It is likely that a combination of processes will be required to deal with the majority of waters, for example filtration followed by UV to remove particles and inactivate microorganisms.
5.2 Multiple barriers
Virtually all water sources require treatment prior to consumption to ensure that they do not present a health risk to the user. Health risks from poor quality water will often be due to microbiological or chemical contamination. Absence of microbiological contamination is generally the most important to human health as pathogens can lead to infectious diseases. Chemical contamination, with the exception of a few substances such as cyanide, tend to represent a more long term health risk. Substances in water which affect its appearance, odour or taste may make water objectionable to consumers and lead to rejection on aesthetic grounds. As microorganisms can be associated with particles and turbidity in water, physical contamination may also represent a health risk as it makes disinfection more difficult.
Most treatment systems are designed to remove microbiological contamination and those physical constituents, such as suspended solids (turbidity) that affect aesthetic acceptability or prevent effective disinfection. A final disinfection stage is nearly always included at the end of the treatment process to inactivate any remaining microorganisms. When a persistent disinfectant, such as chlorine, is applied this also provides a residual that will act as a preservative to prevent biological regrowth during storage and/or distribution in larger systems.
Treatment processes are based on the physical removal of contaminants through filtration, settling (often aided by some form of chemical addition) or biological removal of microorganisms. Usually, treatment consists of a number of stages, with initial pre-treatment by settling or pre-filtration through coarse media, filtration followed by chlorination. This is called the multiple barrier principle.
The multiple barrier approach is an important concept as it provides the basis for effective treatment of water and allows each individual process stage to treat water to a suitable quality for subsequent downstream processes (e.g. filtration can prepare water to ensure it is suitable for UV disinfection). Effective treatment of a supply does not therefore rely on one key stage or process.
The multiple barrier principle applies throughout the supply from catchment all the way to the consumer’s tap. Proper selection and protection of water sources are of prime importance in the provision of safe drinking water. The subsurface is often an effective medium for attenuating contaminants present in the catchment while the design and good maintenance of the well, borehole, spring or intake can help exclude localised pollution from surface runoff. It is always better to protect water from contamination than to treat it after it has been contaminated. Effective source protection, careful choice of aquifer or water intake and well designed and maintained abstraction structures all constitute effective barriers in the multiple barrier principle.
5.3 Coagulation and flocculation
Coagulation and flocculation are used to remove colour, turbidity, algae and other microorganisms from surface waters. The addition of a chemical coagulant to the water causes the formation of a precipitate, or floc, which entraps these impurities. Iron and aluminium can also be removed under suitable conditions. The floc is separated from the treated water by sedimentation and/or filtration, although flotation processes may be used in place of sedimentation.
The most commonly used coagulants are aluminium sulphate and ferric sulphate, although other coagulants are available. Coagulants are dosed in solution at a rate determined by raw water quality near the inlet of a mixing tank or flocculator. The coagulant is rapidly and thoroughly dispersed on dosing by adding it at a point of high turbulence. The water is allowed to flocculate and then passes into the sedimentation tank (sometimes known as a clarifier) to allow aggregation of the flocs, which settle out to form sludge. This sludge will need to be periodically removed.
The advantages of coagulation are that it reduces the time required to settle out suspended solids and is very effective in removing fine particles that are otherwise very difficult to remove. Coagulation can also be effective in removing many protozoa, bacteria and viruses.
The principal disadvantages of using coagulants for treatment of small supplies are the cost and the need for accurate dosing, thorough mixing and frequent monitoring. Coagulants need accurate dosing equipment to function efficiently and the dose required depends on raw water quality that can vary rapidly. The efficiency of the coagulation process depends on the raw water properties, the coagulant used and operational factors including mixing conditions, temperature, coagulant dose rate and pH value. The choice of coagulant and determination of optimum operating conditions for a specific raw water are normally determined by bench scale coagulation tests.
Thus, while coagulation and flocculation are the most effective treatment for removal of colour and turbidity they may not be suitable for small water supplies because of the level of control required and the need to dispose of significant volumes of sludge.
5.4 Sedimentation
Simple sedimentation (i.e. unassisted by coagulation) may be used to reduce turbidity and solids in suspension. Sedimentation tanks are designed to reduce the velocity of flow of water so as to permit suspended solids to settle under gravity. There are many different designs of tanks and selection is based on simple settlement tests or by experience of existing tanks treating similar waters. Without the aid of coagulation, these will only remove large or heavy particles, and due to the length of time this process will take, the system will usually require storage tanks to balance peaks and troughs in demand.
Sedimentation tanks are usually rectangular with length to width ratios between 2:1 and 5:1. The depth of the tank is usually between 1.5 and 2.0m. The inlet and outlet must be at opposite ends of the tank. The inlet should be designed to distribute the incoming flow as evenly as possible across the tank width and to avoid streaming which would otherwise reduce sedimentation efficiency. Baffles may be installed to prevent short circuiting. The outlet should be designed to collect the clarified water over the entire tank width. The tank should be covered to prevent contamination and ingress. Sedimentation tanks require cleaning when performance deteriorates. This will not normally be more frequent than once per year.
5.5 Filtration
Turbidity and algae are removed from raw waters by screens, gravel filters, slow sand, rapid gravity filters or cartridge filters. The difference between slow and rapid sand filtration is not a simple matter of the speed of filtration, but in the underlying concept of the treatment process. Slow sand filtration is essentially a biological process whereas rapid sand filtration is a physical treatment process. Many small private water supplies will rely on cartridge filters consisting of a woven or spun filter within a standard housing.
5.5.1 Screens
Screens are effective for the removal of particulate material and debris from raw water and are used on many surface water intakes. Coarse screens will remove weeds and debris while band screens or microstrainers will remove smaller particles including fish and may be effective in removing large algae. Microstrainers are used as a pre-treatment to reduce solids loading before coagulation or subsequent filtration. A microstrainer may consist of a rotating drum fitted with very fine mesh panels, or may be a fixed mesh that the water flows through. The mesh will ensure that suspended solids, including algae, are retained. The extent of solids removal will be determined by the mesh size and the nature of the raw water. Water will need subsequent treatment downstream and screens should only be considered as a preliminary treatment stage.
5.5.2 Gravel filters
Gravel filters may be used to remove turbidity and algae. A simple gravel filter for the protection of a stream or river inlet is described in Section 3.3.1. A larger gravel filter may consist of a rectangular channel or tank divided into several sections and filled with graded gravel (size range 4 to 30mm). The raw water enters through an inlet distribution chamber and flows horizontally through the tank, encountering first the coarse and then the finer gravel. The filtered water is collected in an outlet chamber. Solids removed from the raw water accumulate on the floor of the filter. Gravel filters can operate for several years before cleaning becomes necessary. The size of a gravel filter will depend on water quality, flow rate and size of gravel. A filter can be up to 12m long, 2 to 5m wide and 1 to 1.5m deep. The filter should normally be sized for a flow rate of between 0.5 to 1.0 cubic metres per square metre of filter surface area per hour (m3/m2.h). A gravel filter will need subsequent treatment downstream, and should only be considered as a preliminary treatment stage.
5.5.3 Slow sand filters
Slow sand filters, sometimes preceded by microstrainers or coarse filtration, are used to remove turbidity, algae and microorganisms. Slow sand filtration is a simple and reliable process and is therefore often suitable for the treatment of small supplies provided that sufficient land is available. Slow sand filters usually consist of tanks containing sharp sand (size range 0.15-0.30mm) to a depth of between 0.5 to 1.5m. For small supplies, modular units of 1.25m diameter are available – a tandem installation would occupy a concrete apron of about 8 to 10 m2. The raw water flows downwards and turbidity and microorganisms are removed by filtration in the top few centimetres of the sand. A biological layer of sludge, known as the schmutzdecke, develops on the surface of the filter that can be effective in removing microorganisms. Treated water is collected in underdrains or pipework at the bottom of the filter. The top few centimetres of sand containing the accumulated solids are removed and replaced periodically. Filter runs of between 2 and 10 weeks are possible, depending on raw water quality and flow rate. Slow sand filters are often operated in tandem; one in service whilst the other is cleaned and time allowed for the schmutzdecke to re-establish.
A variant of the slow sand filter, the “Inverness filter”, has been widely used in Scotland. It uses the same grade of sand and operates at the same flow rate as the traditional slow sand filter but the water flows upwards. Filtration is achieved throughout the filter bed and a true schmutzdecke does not develop. The sand is “washed” by opening a valve at the bottom of the filter and allowing the filter bed to drain rapidly.
Slow sand filters should be sized for a water flow rate of between 0.1 and 0.3 m3/m2.h. The flow rate should be controlled and the filter designed with a treated water reservoir of sufficient capacity to accommodate fluctuations in demand, and thus permit operation of filters at a steady and continuous rate.
5.5.4 Rapid gravity filters
Rapid gravity filters are most commonly used to remove floc from coagulated waters. They may also be used to remove turbidity, algae and iron and manganese from raw waters. Granular activated carbon media may be used to remove organic compounds.
Rapid gravity sand filters usually consist of rectangular tanks containing silica sand and/or anthracite media (size range 0.5 to 1.0 mm) to a depth of between 0.6 and 1.0m. The water flows downwards and solids become concentrated in the upper layers of the bed. Treated water is collected via nozzles in the floor of the filter. The accumulated solids are removed periodically by backwashing with treated water, usually preceded by scouring of the media with air. Frequency of backwashing depends on loading rate and raw water quality and is typically every 24 hours. Backwashing can be initiated automatically after a predetermined headloss has been reached or may be carried out manually. Backwash flowrates will be stipulated by the manufacturer. It is important to achieve the required bed expansion in order to ensure filters are washing properly, but care must be taken to prevent loss of media with too high a flowrate. A dilute sludge that requires disposal is produced which may be discharged to sewer, soak away, or after treatment, to a watercourse provided that any required discharge consent is obtained.
5.5.5 Pressure filters
Pressure filters are sometimes used where it is necessary to maintain hydraulic head in order to eliminate the need for additional pumping. The filter bed is enclosed in a cylindrical shell. Small pressure filters capable of treating up to approximately 15m³/h can be manufactured in glass reinforced plastics. Larger pressure filters are manufactured in specially coated steel. Operation and performance are generally as described for the rapid gravity filter (see Section 5.5.4) and similar facilities are required for backwashing and disposal of the dilute sludge. A similar range of contaminants can be removed depending on the filter medium.
5.6 Aeration
Air stripping is used for removal of volatile organics (e.g. solvents), carbon dioxide, disinfection by-products, some taste and odour causing compounds, and radon (Section 7.1). It is a fairly specialist technique, and not commonly found as a treatment process on private water supplies, although aeration can sometimes be found in the oxidation stage of the treatment process for the removal of iron and manganese (Section 5.7.2)
Aeration processes are designed to achieve efficient mass transfer of oxygen into water and removal of gases and volatile compounds by air stripping. Oxygen transfer can usually be achieved using a simple cascade or diffusion of air into water, without the need for elaborate equipment. Stripping of gases or volatile compounds, however, may require specialised plant that provides a high degree of mass transfer.
For oxygen transfer, cascade or step aerators are designed so that water flows in a thin film to achieve efficient mass transfer. Cascade aeration may introduce a significant headloss; design requirements are between 1.0 and 3.0m to provide a loading of 10 to 30m3/m2.h. If such headloss is unacceptable the alternative is to use compressed air diffused through a system of submerged perforated pipes. These types of aerator are used for oxidation and precipitation of iron and manganese.
To achieve air stripping various techniques can be used including counter current cascade aeration in packed towers, diffused aeration in basins and spray aeration. Packed tower aerators are most commonly used because of their high energy efficiency and compact design.
5.7 Chemical treatment
5.7.1 Control of pH
The pH value of water may need to be adjusted during treatment and before distribution for several reasons, including:
- to ensure that the pH value meets the water quality standards
- to control corrosion in the distribution system and consumers’ installations or to reduce plumbosolvency
- to improve the effectiveness and efficiency of disinfection
- to facilitate the removal of iron and manganese
- to facilitate the removal of colour and turbidity by chemical coagulation
- For removal of hardness
- For removal of other contaminants including some metals
Many raw surface waters are slightly acidic and coagulation processes further increase acidity. Increase of pH can be achieved by:
- dosing with sodium hydroxide, calcium hydroxide or sodium carbonate
- passage of the water through a bed of alkaline medium
- removal of excess carbon dioxide by aeration
Where necessary, reduction of pH can be achieved by dosing with a suitable acid such as sulphuric acid, hydrochloric acid, sodium hydrogen sulphate or carbon dioxide.
5.7.2 Iron and manganese removal
In groundwater, iron is usually present as dissolved ferrous compounds. To remove iron in this form, it is necessary to oxidise ferrous iron, usually by aeration, to the insoluble ferric hydroxide and to remove the precipitated material in a subsequent filtration stage. It is important to ensure that oxidation does not give rise to colloidal species which may pass through the filters. If the iron is present as an organic complex, a strong oxidant such as chlorine or potassium permanganate must be used. Manganese is usually present as dissolved manganous compounds. Removal is achieved by oxidation to insoluble manganese dioxide using catalytic filters or potassium permanganate followed by filtration, or by coagulation at high pH followed by filtration.
In surface waters, iron and manganese are usually present in their oxidised forms and are associated with the suspended solids, which can be removed by filtration. Where coagulation is practised for the removal of colour and turbidity, iron removal may be achieved simultaneously. Iron and manganese may be combined with organic matter in very stable forms. The usual treatment in this case is coagulation followed by oxidation with chlorine or potassium permanganate and filtration.
There are a number of proprietary systems on the market which will oxidise and filter iron and manganese within a single unit with automated control systems.
5.7.3 Taste and odour removal
Taste and odour can be removed by several methods, including aeration, ozonation and adsorption on activated carbon. The method used will depend on the source of the taste and odour. Adsorption on activated carbon is generally the most effective method for the removal of earthy or mouldy taste and odour. Powdered activated carbon can be dosed directly to the water before coagulation and then subsequently removed by sedimentation. Powdered activated carbon is generally used as a one off treatment. Where regular treatment is required, granular activated carbon (GAC) is the preferred solution and this may be used as a filter medium replacing sand in existing filters or alternatively in a post-filtration adsorption stage. In this arrangement, GAC will need to be periodically removed and regenerated, often by the manufacturer and typically once every 12-24 months. When returned and reinstalled it will require washing and running to waste before being returned to supply. This is to allow any contaminants and impurities to be washed out. In this type of installation it is customary to have a system with several GAC beds so that one can be taken out of service without impacting on overall water quality. In private water supplies, the usual method is to use a replaceable GAC cartridge located within a standard cartridge filter housing. This cartridge will be replaced at regular intervals.
5.7.4 Nitrate removal
Nitrate removal is usually achieved by ion-exchange. Water is passed through a column of synthetic resin beads that remove anions including nitrate and exchange them for equivalent amounts of chloride. When the capacity for exchange is exhausted, the resin is regenerated by backwashing with a concentrated solution of sodium chloride. This restores the resin to its initial chloride form. The bed is then rinsed with clean water and returned to service. The waste solution and rinse waters, containing high concentrations of sodium chloride, as well as nitrate, are collected for disposal.
Conventional anion exchange resins have a higher affinity for sulphate than for nitrate. This means that they preferentially remove sulphate and reduce the capacity for nitrate, leading in turn to higher running costs (for regenerant) and greater volumes of waste for disposal. As a result, nitrate selective resins, which give better uptake of nitrate in the presence of sulphate and reduce process costs, are preferred.
Nitrate-selective resins preferentially remove nitrate and also add less chloride to the treated water because of the lower sulphate removal. This is desirable since high chloride concentrations and chloride to bicarbonate ratios are associated with increased corrosion of certain metals. A sodium bicarbonate rinse can be used after regeneration with sodium chloride to convert the resin in the lower part of the bed to the bicarbonate form and reduce the chloride to bicarbonate ratio during the early part of the run.
An ion-exchange plant consists of two or more reactors operated in parallel. Run lengths of up to 24 hours can be achieved before regeneration is necessary. Regeneration consumes up to 2 percent of the volume of treated water and takes about two hours. Performance is affected by the choice of resin, the concentrations of nitrate and sulphate in the raw water, and the volume and concentration of sodium chloride solution used for regeneration. Operation of an ion-exchange plant is normally fully automatic.
Surface waters may require pre-treatment by coagulation to remove organic colour and suspended solids, which would foul the resin.
Nitrate can also be removed by some membrane processes and by biological denitrification. Membrane processes are described in Section 5.8. Resins are available for removal of many anions and cations and have been successfully used at a small scale for removal of colour.
5.8 Membrane processes
The membrane processes of most significance in water treatment are reverse osmosis, ultrafiltration, microfiltration and nanofiltration. These processes have traditionally been applied to the production of water for industrial or pharmaceutical applications but are now being applied to the treatment of drinking water. Their characteristics are illustrated in Figure 12.
Figure 12: Characteristics of membrane processes

If two solutions are separated by a semipermeable membrane, i.e. a membrane that allows the passage of solvent but not of the solute, the solvent will pass from the lower concentration solution to the higher concentration solution. This process is known as osmosis. It is possible, however, to force the flow of solvent in the opposite direction, from the higher to the lower concentration, by increasing the pressure on the higher concentration solution. The required pressure differential is known as the osmotic pressure and the process as reverse osmosis.
Reverse osmosis results in the production of a treated water stream and a relatively concentrated waste stream. Typical operating pressures are in the range 15 to 50 bar depending on the application. Membrane pore sizes are less than 0.002µm. The most common application of reverse osmosis is desalination of sea water although the use of reverse osmosis for nitrate removal has also been proposed. In the case of private water supplies, reverse osmosis units sold as point of use devices are probably the most common type of membrane process encountered.
Ultrafiltration is similar in principle to reverse osmosis, but the membranes have much larger pore sizes (typically 0.002 to 0.03µm) and operate at lower pressures. Ultrafiltration membranes reject organic molecules of molecular weight above 800 and usually operate at pressures less than 5bar.
Microfiltration is a direct extension of conventional filtration into the sub-micron range. It is capable of sieving out particles greater than 0.05µm and will remove most bacteria and amoeboid cysts. It has been used for water treatment in combination with coagulation or powdered activated carbon (PAC) to remove viruses, bacteria, dissolved organic carbon and to improve permeate flux. Microfiltration membranes have pore sizes typically in the range 0.01 to 12µm and do not separate molecules but reject colloidal and suspended material at operating pressures of 1 to 2bar.
Nanofiltration uses a membrane with properties between those of reverse osmosis and ultrafiltration membranes; pore sizes are typically 0.001 to 0.01µm. Nanofiltration membranes allow monovalent ions such as sodium or potassium to pass but reject a high proportion of divalent ions such as calcium and magnesium and organic molecules of molecular weight greater than 200. Operating pressures are typically about 5bar. Nanofiltration may be effective for the removal of colour and organic compounds.
Membrane processes can provide adequate removals of pathogenic bacteria, Cryptosporidium, Giardia, and potentially, human viruses and bacteriophages. However, they should not be relied upon as the sole means of disinfection as in the case of the types of systems encountered in private supplies there is no simple means to check membrane integrity to warn of potential breakthrough of microorganisms.
5.9 Disinfection
Contamination by sewage or animal faeces is the greatest danger associated with water for drinking. This is because sewage from human or animal sources may contain the causative organisms of many communicable diseases. The use of disinfection to kill or inactivate pathogenic microorganisms is necessary if the raw water contains such organisms.
Surface waters including those feeding springs and shallow wells may contain between a few tens of E. coli per 100ml in a source derived from a protected upland catchment to many thousands of E. coli per 100ml in a source derived from a lowland river containing treated sewage effluents. Groundwater is generally less microbiologically active, although contamination may occur through geological features like swallow holes, fissures or through poor construction and protection of borehole headworks.
Several disinfection methods are used in water treatment. Disinfection with chlorine is the most widely used method for large water supplies but its application is less common in small supplies. Ultraviolet irradiation is the most common disinfection method found in private supplies.
Different microorganisms have different susceptibilities to disinfectants, and disinfectants vary in their potency. For a given microorganism, disinfection efficiency is affected especially by disinfectant concentration and contact time, and also by the disinfectant demand of the water, pH and temperature. The product of disinfectant concentration (C in mg/l, measured at the end of the contact period) and time (t in minutes) is called Ct (in mg/l.min) and is an expression of exposure to the disinfectant:
Ct = C × t
The greater the Ct value, or exposure, the more effective disinfection is. Either concentration or contact time, or both, can be manipulated to obtain a desired Ct value. Values of Ct can be useful for comparing the efficiency of disinfectants; the lower the value of Ct to attain a given kill of microorganisms, the more effective the disinfectant. The Ct value can also be used to rank the relative susceptibility of different microorganisms; the higher the Ct value necessary to achieve a given level of kill the more resistant the microorganism.
In the case of ultraviolet irradiation Ct cannot be calculated in the same way and the exposure is expressed as UV radiation energy density, which is equivalent to (power × time) per unit area, expressed in milliwatt seconds per square centimetre (mW.s/cm2) or millijoules per square centimeter (mJ/cm2).
5.9.1 Ultraviolet irradiation
Ultraviolet (UV) irradiation is the preferred method for disinfection of small supplies with small distribution networks or retention time. Chlorination may be more suitable for larger schemes in which it is necessary to maintain a residual disinfectant during storage and distribution. UV disinfection efficiency is particularly affected by water quality and flow rate. The water to be disinfected must be of good quality and particularly low in colour and turbidity. The usual measure for the suitability of treatment by UV disinfection is UV254 absorbance, which may be measured with online monitors or sampled and analysed at a laboratory. UV254 can often vary with levels of colour and organic matter in the water and manufacturers of UV disinfection equipment will be able to advise on the suitability for particular waters. However, prefiltration is almost always required prior to UV disinfection, especially if Cryptosporidium is likely to be present.
Special lamps are used to generate UV radiation; they are enclosed in a reaction chamber made of stainless steel or, less commonly, plastics. Low pressure mercury lamps, which generate 85% of their energy at a wavelength of 254 nm, are most commonly used. Their wavelength is in the optimum germicidal range of 250 to 265 nm. These lamps are similar in design, construction and operation to fluorescent tubes except that they are constructed of UV transparent quartz instead of phosphorcoated glass. The optimum operating temperature of the lamp is around 40°C so the lamp is normally separated from the water by a sleeve to prevent cooling by the water. The intensity of UV radiation emitted decreases with lamp age. Typical lamp life is about 10 to 12 months, after which the output is about 70% of that of a new lamp. Manufacturers will advise on lamp maintenance requirements. It is worth noting that visually a lamp will still appear the same, even when output has decreased, and so the fact that a lamp is emitting light is not necessarily a guarantee that it is delivering the required dose.
The usual UV reactor configuration comprises a quartz sleeved low pressure mercury lamp in direct contact with the water. Water enters the unit and flows along the annular space between the quartz sleeve and the wall of the chamber. Other configurations include lamps separated from the water, for example, those were the lamps are surrounded by bundles of PTFE tubes through which the water flows.
Disinfection will only be effective provided that a sufficient dose of UV is applied. The dose of UV radiation is expressed as an energy flux, in units of mW.s/cm2 (milliwatt seconds per square centimetre), or more commonly as millijoules per centimeter squared (mJ/cm2) which is the product of the intensity given out by the lamp and the residence time of water in the reactor. The minimum dose required for disinfection depends on several factors, including the susceptibility of microorganisms but is generally taken to be around 40mJ/cm2.
It is important, to ensure effective disinfection, that both residence time and UV intensity are adequate. UV intensity will be diminished by ageing of the lamp, fouling of the lamp sleeve by deposits, and absorption of UV radiation by water contaminants such as natural colour. For these reasons lamps need to be changed at the recommended intervals and the quartz sleeve may require periodic cleaning. Some units incorporate a manual wiper for cleaning whilst others incorporate automatic mechanical cleaning.
Colour and turbidity will both affect radiation intensity in the reactor and turbidity may protect microorganisms from the radiation. The water to be treated should be tested for transmissivity or absorbance (UV254) by the manufacturer or supplier in order to estimate worst-case transmission values and to adjust contact time accordingly. More advanced units incorporating UV monitors have the facility to automatically adjust the energy input to the UV lamp to achieve the required UV intensity.
Unlike chlorination, UV is effective in inactivating Cryptosporidium provided that a sufficient UV dose is applied. However, where Cryptosporidium is likely to be present and its removal is required then pre-filtration capable of achieving a turbidity of less than 1NTU is required prior to UV disinfection. Pre-filtration provides an additional barrier to passage of oocysts into the treated water removes particles that shield microorganisms from the UV light and helps to reduce fouling of the UV lamp.
UV irradiation equipment is compact and simple to operate. Maintenance requirements are modest, although specific systematic maintenance is essential. Other advantages include short contact time and the absence of any known byproducts of significance to health. The principal disadvantage is the absence of any residual effect, necessitating careful attention to hygiene in the storage and distribution system.
The build-up of scale on the sleeves of the lamps will eventually reduce their transmittance and they must be cleaned or replaced regularly. Some units have UV intensity monitors and alarms which provide a continuous check on performance and these are strongly recommended. These devices may prevent the flow of water if the required intensity of UV radiation is not achieved, for example when the lamps are warming up or because of scale formation. UV intensity monitors may not be available on smaller units and it is therefore essential that the manufacturer’s instructions regarding lamp warmup, cleaning and replacement are followed to ensure optimal performance.
Lamp replacement is usually a simple operation but may involve a significant downtime for reactors with many lamps. This difficulty may be overcome by use of multiple units or by having a treated water storage tank capable of maintaining supply whilst maintenance is carried out.
There are many UV units on the market and care must be taken when selecting a unit for a private water supply. Units must be designed for drinking water treatment, and where possible, validated units are to be recommended. These will have been tested to ensure that the stated level of disinfection is achieved under normal operating conditions. UV units for ponds and fish tanks etc. are not suitable for use on supplies for human consumption.
5.9.2 Chlorine Chemistry
Chlorine, whether in the form of pure chlorine gas, sodium hypochlorite or calcium hypochlorite, dissolves in water to form hypochlorous acid (HOCl) and hypochlorite ion (OCl–). For example, chlorine gas dissolves rapidly in water, initially forming hypochlorous and hydrochloric acids:
Cl2 + H2O ⇔ HOCl + HCl
Hypochlorous acid is a weak acid which undergoes partial dissociation to produce a hydrogen ion (H+) and a hypochlorite ion (OCl–):
HOCl ⇔ H+ + OCl–
The total concentration of chlorine, hypochlorous acid and hypochlorite ions is referred to as the free available chlorine. If ammonia is present in the raw water, the hypochlorous acid can react to produce chloramines. The total concentration of the chloramines and any organic nitrogen chlorine containing compounds is referred to as the combined available chlorine. Combined available chlorine is a less powerful disinfectant than free available chlorine but gives a more persistent residual.
The formation of combined chlorine is due to a sequence of reactions whereby hydrogen in ammonia is progressively replaced by chlorine as follows:
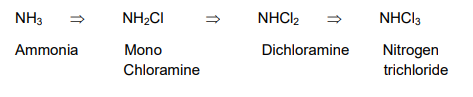
If a large chlorine dose is applied (relative to ammonia), as is practiced in breakpoint chlorination, then nitrogen is formed.
2NH2Cl + Cl2 ⇒ N2 + 4HCl
The effectiveness of chlorine for disinfection depends on the form of chlorine, its concentration and the contact time. Hypochlorous acid is a more powerful disinfectant than the hypochlorite ion and chlorination is usually practiced at values of pH favourable to its formation. The World Health Organization recommends that for the effective disinfection of drinking water “the pH should preferably be less than 8.0 and the contact time greater than 30 minutes, resulting in a free chlorine residual of 0.2 to 0.5mg/l”. Figure 13 below shows how the equilibrium between hypochlorous acid and the hypochlorite ion shifts towards the hypochlorite ion as pH increases.
Chlorination processes need to be carefully controlled in order to minimise the formation of taste and odour forming compounds. There may also be a need to control the formation of disinfection by-products including THMs. Therefore, for small supplies, consideration should be given to using alternatives to chlorination, such as UV.
Figure 13: hypochlorous acid and hypochlorite ion equilibrium

5.9.3 Sources of chlorine
Chlorination can be achieved by using liquefied chlorine gas, sodium hypochlorite solution or calcium hypochlorite granules. Chlorine gas is very reactive and highly toxic and must be carefully stored and handled. It is used for treatment of large public supplies but the inherent danger of using chlorine gas has resulted in an increased use of sodium hypochlorite or the electrolysis of brine (electro-chlorination) as alternative sources of chlorine.
The use of chlorine gas for treatment of small water supplies is not recommended. Gas chlorination is generally not appropriate for supplies of less than 10m3/d or where the available head is less than about 4.0m. Leaks of chlorine gas are very dangerous. A separate area is necessary for storage of chlorine gas and an alarm system to detect leakage of chlorine must be installed. It is common practice to install alarm systems to indicate failure of the chlorine injector system or carrier water flow.
Liquefied chlorine gas is supplied in pressurised containers. The gas is withdrawn from the cylinder and is dosed into water by a chlorinator, which both controls and measures the gas flow rate.
Sodium hypochlorite solution (14 to 15% by mass (m/m) available chlorine) can be delivered to site in drums. No more than one month’s supply should be delivered at one time, as its decomposition (particularly on exposure to light) results in a loss of available chlorine and an increase in concentration of chlorate, relative to chlorine.
Alternatively, sodium hypochlorite solution (0.5 to 1.0% mass per volume (m/V) available chlorine) can be generated on site by the electrolysis of brine (sodium chloride solution). A typical electro-chlorination system consists of a water softener, a salt saturator, a voltage rectifier, an electrolysis cell and a storage tank containing up to three day’s supply of hypochlorite solution. Hydrogen, which is produced during electrolysis, must be vented safely. These systems are compact and eliminate the need to store and handle the toxic and corrosive chlorination chemicals.
There is a wide choice of equipment available for dosing sodium hypochlorite solution. Simple gravity fed systems in which sodium hypochlorite solution is dripped at a constant rate into a tank of water have been used successfully and have proven reliable provided that the rate of flow into supply and the chlorine demand of the water are constant. Where the flow is more variable, water powered hypochlorinators that adjust the flow of sodium hypochlorite proportionately to the flow of water may be suitable. Electric dosing pumps can operate under flow proportional or chlorine residual control and thus maintain a consistent chlorine residual under conditions of variable flow or chlorine demand.
Calcium hypochlorite can be supplied in powdered, granular or tablet form (65 to 70% m/m available chlorine). Calcium hypochlorite is stable when dry and several month’s supply can be stored. It will however react with moisture in the air to form chlorine gas. Calcium hypochlorite dosing equipment is relatively simple. Most allow calcium hypochlorite to dissolve in a known volume of make-up water, which is then mixed with the main supply. Tablets are most commonly used, as their rate of dissolution is predictable. Control of dosage (proportional to the rate of dissolution) is often limited to changing the depth of immersion of the tablets in the make-up water or to changing the proportion of the make-up water to total flow.
5.9.4 Methods of chlorination
Several regimes of chlorination can be used, including marginal (simple) chlorination, breakpoint chlorination, superchlorination/dechlorination and chloramination. On small supplies, it is probable that only marginal chlorination would be used in most cases. Marginal chlorination involves the dosing of chlorine to produce a suitable residual free available chlorine concentration.
Breakpoint chlorination can and is used for removal of ammonia. Sufficient chlorine is added to exceed the demand for chloramine production and to ensure a free available chlorine residual. The chlorine dose must be carefully controlled to avoid forming dichloramine and nitrogen trichloride which can cause taste and odour problems. Breakpoint chlorination requires a dose of around 10 mg/l chlorine dosed per mg/l ammonia removed. The actual dose depends on water quality and has to be determined for each water.
The resultant free available chlorine residual should remain in the range 0.2 to 0.5mg/l. It is recommended that the contact time should be at least 30 minutes. The design of the contact system is very important. Applied chlorine must be mixed rapidly with the water and then passed through a contact tank, ideally with baffles or a serpentine arrangement to prevent short circuiting or retention in dead zones.
5.9.5 Control of chlorination
Chlorine residual control is the most common method of control where chlorine is dosed continuously into the water. If the quality of the water and hence the chlorine demand varies appreciably, it is necessary to use a control system to maintain a constant chlorine residual. A sample of chlorinated water is withdrawn downstream of the chlorination system and the chlorine residual in the treated water is monitored continuously. The signal from the chlorine analyser system is used to adjust the chlorine dose thus maintaining the required residual chlorine concentration. Where water quality is consistent, constant rate control or flow proportional control may be appropriate. In the former, a constant dose of chlorine is applied and in the latter a chlorine dose proportional to the flow of water is applied automatically under control of a signal from the flow sensor. There is no standard for chlorine in the regulations, but excess levels give rise to customer complaints of taste and odour.
5.10 Corrosion control
Corrosion is the partial dissolution of the materials constituting the treatment and supply systems, tanks, pipes, valves, and pumps. It may lead to structural failure, leaks, loss of capacity, and deterioration of chemical and microbiological water quality. The internal corrosion of pipes and fittings can have a direct impact on the concentration of some water constituents, including lead, copper and nickel. Corrosion control is therefore an important aspect of the management of a water supply system.
Corrosion control involves many parameters, including the concentrations of calcium, bicarbonate, carbonate, and dissolved oxygen, as well as pH. The detailed requirements differ depending on water quality and for each distribution system material. The pH controls the solubility and rate of reaction of most of the metal species involved in corrosion reactions. It is particularly important in relation to the formation of a protective film at the metal surface. For particular metals, alkalinity (carbonate and bicarbonate) and calcium (hardness) also affect corrosion rates.
5.10.1 Concrete and cement
Concrete is a composite material consisting of a cement binder in which an inert aggregate is embedded. Cement is primarily a mixture of calcium silicates and aluminates together with some free lime. Cement mortar, in which the aggregate is fine sand, is used as a protective lining in iron and steel water pipes. In asbestoscement pipe, the aggregate is asbestos fibres. It should be noted that research has indicated that the use of asbestos in cements in water mains does not pose a health risk. Cement is subject to deterioration on prolonged exposure to aggressive water, due either to the dissolution of lime and other soluble compounds or to chemical attack by aggressive ions such as chloride or sulphate and this may result in structural failure. Aggressiveness to cement is related to the “Aggressivity Index”, which has been used specifically to assess the potential for the dissolution of concrete. A pH of 8.5 or higher may be necessary to control cement corrosion.
5.10.2 Copper
Copper tubing may be subject to general corrosion, impingement attack and pitting corrosion. General corrosion is most often associated with soft, acid waters; waters with pH below 6.5 and hardness of less than 60 mg/l CaCO3 are very aggressive to copper. Impingement attack is the result of excessive flow velocities and is aggravated in soft water at high temperature and low pH. The pitting of copper is commonly associated with hard groundwater having a carbon dioxide concentration above 5mg/l and high dissolved oxygen. Surface waters with organic colour may also be associated with pitting corrosion. A high proportion of general and pitting corrosion problems are associated with new pipe in which a protective oxide layer has not yet formed.
5.10.3 Lead
Lead corrosion (plumbosolvency) is of particular concern. Lead piping is still common in old houses, and lead solders have been used widely for jointing copper tube. The solubility of lead is governed by the formation of insoluble lead carbonates. The solubility of lead increases markedly as the pH is reduced below 8 because of the substantial decrease in the equilibrium carbonate concentration. Thus, plumbosolvency tends to be at a maximum in waters with a low pH and low alkalinity, and a useful interim control procedure pending pipe replacement is to maintain pH in the range 8.0 to 8.5 and possibly to dose orthophosphate.
5.10.4 Nickel
Concentrations of nickel up to around 1 mg/l may arise due to the leaching of nickel from new nickel-chromium plated taps and from stainless steel pipes and fittings. Nickel leaching reduces over time. Increase of pH to control corrosion of other materials should also help to reduce leaching of nickel.
5.10.5 Water treatment for corrosion control
To control corrosion in water distribution networks the methods most commonly applied are adjusting pH, increasing the alkalinity and/or hardness, or adding corrosion inhibitors such as sodium polyphosphates or silicates and orthophosphate. The quality and maximum dose to be used should be in line with appropriate national specifications for such water treatment chemicals. Although pH adjustment is an important approach its possible impact on other aspects of water supply technology, including disinfection, must always be taken into account.
Treatment to reduce plumbosolvency usually involves pH adjustment. When the water is very soft (less than 50mg/l CaCO3), the optimum pH is about 8.0 to 8.5. Alternatively, dosing with orthophosphoric acid or sodium orthophosphate might be more effective particularly when plumbosolvency occurs in nonacidic waters. Wherever practicable, lead pipework should be replaced. Grants for pipe replacement may be available from the local authority and some water companies offer a lead pipe replacement service.
6. Point-of-use treatment
A point-of-use device is any form of water treatment apparatus that may be installed or used by a householder within their premises and normally at the point of supply. A point-of-use device may be installed before the householder’s tap (plumbed inline or otherwise attached to the water pipe), connected to the tap by an adapter or separate from the plumbing system (such as a freestanding gravity filter). Thesedevices handle relatively small volumes and it is usual for only water used for drinking and cooking to be treated. The treated water is supplied to a separate tap. It is important that the householder is made aware of the need to use the dedicated drinking water tap. Moreover, point-of-use treatment may not be appropriate if the untreated water would pose a risk to health if used for bathing or washing. In any case, it may be necessary to consider the possibility of providing an alternative supply, rather than attempting to improve a supply of inferior quality.
Some point-of-use devices have been designed and developed to provide additional treatment to mains water, although equipment designed for use with raw water sources is available. Contaminants in a raw water source for a private water supply may be present at higher concentrations than in a public supply and may affect the performance of some point-of-use devices. For example hardness compounds may precipitate on filters and membranes and iron and manganese compounds may deposit on UV tubes. Some manufacturers of point-of-use devices incorporating activated carbon specifically state that their devices should not be used on waters that are microbiologically unsafe or of unknown quality.
The foregoing observations do not rule out the application of point-of-use devices to small water supplies but appropriate pre-treatment may be required and careful attention must be paid to the maintenance of the device. Where there is any doubt as to the effect of water quality on the performance of a point-of-use device, water quality data should be obtained and expert advice on the applicability of the device should be taken.
In practice, there is typically an expectation that a whole supply will be treated, and that point of use devices are not appropriate for treatment of private supplies. However, as part of a Local Authority risk assessment, there may be a recommendation that point of use treatment is an appropriate mitigation for certain risks. This is likely to be for parameters that do not cause an immediate danger to health, such as pesticides, or where health risks are greater for a certain part of the population (e.g. babies and nitrate).
6.1 Particulate filters
There are several types of particulate filters using different media to remove suspended matter from water in the range 0.5 to 50µm, or greater. Particulate filters may be used to reduce turbidity and microorganisms, or to remove specific inorganic particulates such as iron, aluminium or manganese compounds. Many particulate filters are incorporated into proprietary point-of-use devices to protect subsequent processes such as activated carbon filtration, reverse osmosis or UV disinfection.
Filters are made in several forms, for example discs, woven or resin-bonded cartridges and ceramic candles. Filtration is effected by pleated paper and felt, woven cartridge filters manufactured from viscose, polypropylene, fibrillated polypropylene, nylon or fibreglass, non-woven cartridges manufactured from resinbonded polyester, and Kieselguhr ceramic.
Water passes through the filter and particles and microorganisms may be retained depending on the pore size of the filter. Particle removal may be achieved by surface filtration or by retention within the filter material. Some of the cartridge and ceramic filters have graded pore sizes so that larger particles are retained on the surface while smaller particles penetrate the filter where they are retained. As the filter blocks the flow rate of water decreases and this is often the first sign that a filter needs replacing or cleaning. Replacement of a filter is usually recommended after a specific time or after a specific volume of water has been filtered and filtration period will depend on the quality of water being treated. A ceramic candle may additionally require periodic cleaning of the surface. Growth of bacteria on these filters and the possible contamination of the treated water are of concern. Some filters are impregnated with silver to prevent or inhibit the growth of bacteria.
The treatment capacity of filters depends on the water quality, water pressure and the pressure drop across the filter, which in turn is dependent on pore size and porosity. An 18 cm ceramic candle operating under gravity may have an output of 20 litres per day whereas a 13 cm ceramic candle operating at 3 bar may have an output of 150 litres per hour. In hard water areas (greater than about 200 mg/l CaCO3), calcium carbonate may precipitate and block the device. The manufacturer’s advice on suitability should therefore be sought.
6.2 Reverse osmosis units
Reverse osmosis (RO) has been used in recent years for the production of drinking water from low quality raw waters. Large scale RO plants are used for the desalination of seawater to produce drinking water in countries where adequate supplies of fresh water are not available. Because of the high concentrations of dissolved salts in seawater, the osmotic pressure is high and operating pressures up to 70 bar are used. Domestic RO units operate at much lower pressures, as low as 1 bar, because of the typically lower concentration of dissolved salts in the water to be treated. RO systems will remove, to varying degrees, a range of physically and chemically diverse substances including dissolved inorganic species (e.g. sodium, calcium, nitrate and fluoride) and organic pollutants including pesticides and solvents. RO can also produce pathogen free water.
The water to be treated by RO must be of good quality to prevent fouling or scaling of the membrane and a raw water supply will usually require pre-treatment.
Raw water enters the RO unit and treated water flows through the semi-permeable membrane, usually manufactured from polyamide. Some membranes such as cellulose acetate may support bacterial growth and are therefore unsuitable. The flow rate of treated water is very low at the pressures used in a domestic unit and the treated water is collected in a storage tank to buffer supply and demand. Usually a level sensor in the storage tank controls the operation of the RO unit.
The membrane does not become exhausted or saturated although it will require periodic chemical cleaning and replacement. The storage tank must be constructed of an approved material and be protected from contamination. Periodic disinfection of the tank is recommended. There is evidence that inadequate cleaning of the storage system and pipework associated with RO units can result in proliferation of bacteria that are of health significance.
Water treated by reverse osmosis will generally be very soft and likely to increase rates of corrosion in metallic fittings. If used as a point of use device, subsequent downstream plumbing should be kept to a minimum to reduce the risk of ingesting metals. In addition, reverse osmosis water can lower pH and this should be borne in mind when developing monitoring programmes. The lack of minerals and hardness in reverse osmosis water may lead to consumers experiencing taste issues, and remineralisation of water treated by reverse osmosis is recommended where possible.
A further disadvantage of RO is that a relatively high volume of water is wasted. Typically, for each volume of drinking water produced, three volumes must be wasted unless it can be used for no potable purposes such as toilet flushing. RO should be considered if no alternative treatment could make the raw water safe to drink.
6.3 Nitrate removal units
Ion exchange, using nitrate specific anion exchange resins, can be used to remove nitrate ions from water. Water is passed through a bed of anion exchange resin and the anions in the water, including nitrate, are replaced by chloride ions. The resins used are nitrate selective, i.e. nitrate is exchanged in preference to other ions such as sulphate and bicarbonate. The chloride concentration in the treated water will invariably be increased, possibly to above the indicator parameter value of 250mg/l. This could have an adverse effect on the taste of the water but probably would not have implications for health. However, excessive chloride concentrations could lead to corrosion of pipework and fittings.
Ion exchange units are installed inline and the resin is regenerated as required (manually or automatically) with sodium chloride solution (brine). Microbiological growth on the resin could result in the formation of nitrite from nitrate but, as the brine also acts as a disinfectant, this should not be a problem provided that regeneration is performed at recommended intervals. Units incorporating replaceable or disposable cartridges containing resin are also available. Where anion exchange treatment is applied to a source that exhibits unsatisfactory bacteriological quality, it will be necessary to provide a disinfection stage after the resin.
6.4 Adsorption filters
6.4.1 Activated carbon
Activated carbon removes contaminants from water by physical adsorption. Adsorption will be affected by the amount and type of the carbon, the nature and concentration of the contaminant, retention time of water in the unit and general water quality (temperature, pH, etc.). Granular activated carbon (GAC) is the most common medium employed although powdered activated carbon (PAC) and block carbon are also sometimes used. The filter media is contained in replaceable cartridges; a particulate filter at the outlet of the cartridge removes carbon fines from the treated water. Other similar types of filter are pre-coated activated carbon filters and filters using different adsorbents, such as bone charcoal (an adsorbent made from charred animal bones, consisting principally of hydroxyapatite together with about 10% by weight of carbon).
Activated carbon filters will remove (to varying degrees) suspended solids, chlorine and organic contaminants including pesticides, trihalomethanes and some of the humic substances responsible for the yellow to brown coloration commonly found in upland surface waters. The hydraulic retention time is a critical factor in determining the removal of contaminants. In point-of-use devices this is often short and can limit the removal of contaminants, particularly pesticides.
Activated carbon is an ideal medium for the accumulation and growth of microorganisms. Activated carbon removes chlorine from the water and bacterial growth can occur even on filters treating chlorinated water. Thus there is concern that direct consumption of water from activated carbon devices may cause health problems due to bacteria released into the water. Inhalation of bacteria containing aerosols, for example during washing, could also be harmful.
6.5 Water conditioners
6.5.1 Ion-exchange softeners
Although not a necessary treatment to provide safe drinking water, it is sometimes beneficial to remove calcium and magnesium in order to prevent scaling and encrustation with limescale from very hard waters. Softening is achieved by cation exchange, whereby water is passed through a bed of cationic resin and the calcium ions and magnesium ions in the water are replaced by sodium ions. Unlike the carbonates and bicarbonates of calcium and magnesium, sodium carbonates and bicarbonates do not cause scale formation or increased use of soap for washing. When the ion-exchange resin is exhausted, i.e. the sodium ions are depleted, it is regenerated using a solution of sodium chloride.
ater softening could possibly result in a breach of the UK national standard for sodium (200mg/l). Water softeners of this type are not intended for the production of drinking water and softened water should not be used for drinking but may be used for washing. Blending softened and unsoftened water can produce partially softened water, suitable for drinking. In all cases where these devices are installed, a separate unsoftened drinking water supply must be maintained.
The process of de-alkalisation can also soften water. Water is passed through a bed of weakly acidic ion exchange resin and the calcium ions and magnesium ions are replaced by hydrogen ions. The hydrogen ions react with the carbonate and bicarbonate ions to produce carbon dioxide. The hardness of the water is thus reduced without any increase in sodium levels. De-alkalisation resins are available as disposable cartridges. They must be replaced after the interval recommended by the manufacturer, otherwise they become exhausted or will eventually become colonised by bacteria during periods of non-use.
6.5.2 Physical water conditioners
A variety of devices on the market generate magnetic or electrical fields for the water to pass through, or may be intended to release trace concentrations of zinc or other metals. Some of these devices must be plumbed into the pipework, whilst others are non-intrusive and can be simply clamped on or wrapped around the pipework. The effect of these devices can be to physically condition the water. The physical conditioning causes no change to the chemical composition of the water and only exerts a physical effect. Since the chemical composition is not changed, the calcium salts still precipitate when the water is heated or concentrated by evaporation. The effect of the physical conditioning, or presence of zinc, is to cause the calcium salts to precipitate differently such that they are less encrusting. Physical conditioning can produce some of the benefits of softening but without actually removing calcium.
These devices do not treat the water to remove ions, chemicals or remove pathogens and should not be regarded as treatment within the context of providing safe wholesome water as defined by the Private Water Supplies Regulations. If such devices are used to treat water of unsatisfactory bacteriological quality it will be necessary to incorporate a disinfection stage.
6.6 Disinfection units
6.6.1 Ultraviolet irradiation
Ultraviolet (UV) disinfection is discussed fully in Section 5.9.1. Point-of-use UV disinfection units are available for domestic use, which are installed inline. No residual disinfecting capacity is imparted to the treated water so the unit should be located as close as possible to the drinking water tap. UV devices are most effective when the water is of low colour and turbidity. UV devices are often installed together with other upstream treatments, such as filtration, to prepare the water for effective disinfection.
Manufacturers’ recommendations must be followed regarding installation, operation and maintenance. In particular, the maximum design flow rate should not be exceeded, lamps should be allowed to reach their operating temperatures before water is passed through the unit and lamps should be cleaned and replaced as recommended. A continuous UV monitor and an alarm or failsafe device is strongly recommended and although not usually fitted as standard on point-of-use units, they are usually available as an extra.
6.6.2 Chlorination
Chlorination is discussed fully in Section 5.9.2. Chlorination is generally unsuitable for point-of-use treatment.
6.7 Combination devices
Some point-of-use devices require a high quality water in order to operate effectively, for example reverse osmosis units and ultraviolet irradiation units require that the influent water is of very low turbidity. Where such devices are used on small supplies, pre-treatment of the water may be required. Combination devices are designed for this purpose and it is usual for point-of-use devices that require a high quality of water to include pre-treatment units.
6.8 Maintenance requirements
Point-of-use water treatment equipment in general is not fit and forget technology and regular maintenance is essential to ensure the continued supply of safe drinking water. Equipment manufacturers’ maintenance instructions must be followed as a minimum requirement. Ideally maintenance requirements should reflect the raw water quality and flow, but this is often not the case and instructions may be vague. Manufacturers’ maintenance instructions may be based on the assumption that the equipment will be used to treat mains drinking water. Raw waters used for private supplies will always be of worse quality than public supplies and maintenance requirements quoted for treatment of feed water of potable standard will be inadequate. Maintenance requirements must be clear and the consequences of failing to maintain adequately should be highlighted. The majority of devices that fail do so as a result of inadequate maintenance. Special points to observe include:
- Power supplies should be disconnected prior to servicing
- Filter cartridges must be changed on a regular basis. The water supply must be turned off before the housing is unscrewed and the cartridge is removed. The housing should be rinsed out with clean water (only use cleaning materials if specified by the manufacturer) and the new cartridge installed. The old cartridge may be contaminated and must be disposed of safely; precautions should be taken to prevent contamination of the replacement filter. Manufacturers of point-of-use devices should provide, within their instructions for filter units, guidance on the safe handling and disposal of used filter elements.
- UV lamps should be disconnected from the electricity supply and withdrawn carefully. Replacement is simply the reverse operation but care must be taken not to handle the glass. Exposure to UV irradiation must be avoided.
- Intermittent operation may reduce the life of a UV lamp and frequent on/off operation should be avoided (this may invalidate any warranty). Low pressure lamps give out little heat and will not be damaged by operation under no flow conditions. However, scale formation may be greater under these conditions and more frequent cleaning of the quartz sleeve may be required. Again care must be taken when withdrawing and installing the sleeve.
7. Treatment for radon and uranium
7.1 Radon removal
There are three main treatment methods for radon removal, including decay storage, GAC and aeration. Due to the large size of the radon atom, it can also be removed by reverse osmosis and nanofiltration techniques. With all the removal systems, location is a key issue, both for hydraulic reasons and radiation exposure. Some systems may require an additional pump to be installed, or a bypass system. This increases the complexity of the system. Where a system results in the build-up of radioactive substances, it may not be appropriate to keep it under the sink. On the other hand, if the radiation risk means that there is a need to build an extra outbuilding to house a unit, the cost may be prohibitively expensive. For radon removal, point-of-use systems fitted to the drinking water tap (as opposed to point-ofentry systems, which treat the entire supply) are not acceptable as radon is released wherever water is used in the house and it can then be inhaled. Table 2 summarises the features of the principal methods for radon removal.
7.1.1 Decay storage
As radon (222Rn) has a half-life of 3.82 days (i.e. its radioactivity halves every 3.82 days), it is possible (provided that mixing and short-circuiting are avoided) to store it to achieve an adequate reduction in radioactivity. The amount of time required will depend on the level of activity. An eightfold reduction would take two weeks to achieve. For household consumption, this would typically require two 10m3 tanks, used alternately, which is impracticably large for most locations. With a lower activity level, requirements would be less and this option may be feasible.
7.1.2 Granular activated carbon
GAC was first used in the US in 1981 for radon removal. The method has been found to be very effective and is generally quoted as achieving about 95% radon removal. GAC is commonly used for removing taste, colour, odour and synthetic organic chemicals. It works by adsorption and the extremely high internal surface area within the porous structure is responsible for its effectiveness.
The main drawback of GAC is that as the radon is trapped in the filter, the radioactivity of the filter increases. Although radon decays rapidly, there is a continuously increasing radioactivity due to other radionuclides being trapped and the build-up of longer lived radionuclides further down the radon decay chain (notably 210Pb). As such, it is important to either shield the filters or place them in a separate shed outdoors or in an unused basement. There are also disposal problems with these levels and the filters have to be handled with care. With local authority permission, substances up to a radioactivity of 15Bq/g can be disposed of to landfill with other household waste. The time before the filter reaches this level will depend on activity levels of radon in the raw water and on the retention time in the GAC. Once a GAC filter is taken out of service its radioactivity will fall as the adsorbed radon and other radionuclides decay. It has been shown that after three to four weeks out of service the activity of a GAC unit can be close to background levels.
In order to avoid clogging of the filter and to extend its life, it may well be necessary to pre-treat the water. Typically, this would involve a sediment filter and possibly an ion- exchange unit to remove other radionuclides but the treatment required would depend on the levels of other contaminants in the water.
7.1.3 Aeration
Aeration is the preferred treatment for radon removal. In the natural environment this process ensures that most waters coming from springs in radon emitting rocks quickly lose their radon to the atmosphere. The main reason why problems occur with radon in many private supplies is because the water is either abstracted from the rock directly or very soon after. Aeration allows radon to be easily vented to the outside air thus preventing build-up of radiation levels and means there are no disposal issues. As such, the system will typically require less maintenance. Depending on the system, there may be a need for a pressure tank or an additional pump.
7.2 Uranium removal
Unlike radon, uranium does not transfer from water to air once inside houses and thus treatment at point-of-use seems more appropriate than treatment at point-of-entry. Point-of-use treatment has the potential advantage that much smaller volumes require treatment. Many methods are available for removing heavy metals from water and as such there is no shortage of possible solutions to a problem with uranium but ion exchange and reverse osmosis are the only suitable options for private water supplies, the former is normally the preferred method. For ion exchange resins are available that can provide effective treatment systems. Reverse osmosis is also effective, it has the advantage that packaged point-of-use systems are available that can be used without any modifications. Uranium removal is rarely practised so advice should be sought from professional water treatment equipment suppliers or consultants. Table 3 summarises available systems.
Table 2: Radon Removal
Treatment option | Efficiency | Availability | Disposal issues | Approximate cost per household | Other issues |
---|---|---|---|---|---|
Decay storage | Up to 100% | Storage tanks readily available. | Good ventilation system required (or very large tanks). | Variable, depending on size of tank and ventilation system. | Low maintenance. Would need to be designed for each site. Would require further testing and monitoring. Cannot handle higher levels easily. |
GAC specifically designed for radon removal | Variable. Up to >99.9% | Common technology. Many carbon suppliers and suitable apparatus. | Carbon becomes radioactive and high radiation doses reported around filter as well – needs careful placement/shielding. Used filter may need specialist disposal. | £500 – £1,000, assuming no pre-treatment and no new building required. Possible annual filter replacement and high disposal costs. | Widely used in USA. Computer program available for home users (free download from EPA) to assess radiation risks and removal rates. Waste disposal and maintenance are key concerns. A number of available carbons have been specifically tested for radon removal. Not as effective or “safe‟ as aeration. |
GAC – standard water treatment package | Unknown. May be up to 99.9% | Yes – domestic water treatment companies. | As GAC above. | About £2,500. | Provides a complete water treatment system. From initial observations, size of GAC filter too small for effective radon removal. No specific units have quoted radon removal rates. Expensive, and complex waste / maintenance issues. |
Aeration | Up to >99.9% | Widely used technology but not for this application at this scale – companies are able to design and test systems. A few ready- tested ones available. | Adequate ventilation required. | £1,000 upward. Running costs about £20 p/a. | Can operate without pre-treatment, unless water is very hard and needs softening. Some very good package systems available. Low maintenance – one annual clean recommended. With less disposal issues, no radiation build up and reasonable costs, aeration appears to be the best solution. |
Table 3: Uranium Removal
Treatment option | Efficiency | Availability | Disposal issues | Approximate cost per household | Other issues |
---|---|---|---|---|---|
Reverse Osmosis Point-of-use (under sink)
| >95% systems | Package available from domestic water treatment suppliers. | Waste stream unlikely to be a problem. There will be a build up over time on the membrane and to some extent on any preceding GAC filter – this should be monitored. | About £500. Running costs about £40 a year. | Pre-treatment for radon removal required. Possibly a need for shielding of the system, due to build-up on the RO membrane. No direct process guarantees from manufacturers. Regular changing of the membranes and any pre-filters would be required. |
Reverse osmosis Point-of-entry (whole supply) | >95% | Package systems available from domestic water treatment firms. Also available from medical sector. | As above. | About £2,500, with £200 a year running costs | Possible need for shielding / careful location. Again, no direct process guarantees. Regular filter changes required. Generally, treating far more water than is required. |
Ion-exchange Point-of-use (under sink) | >95% (dependent on resin) | Many water softeners available. Suitable resins (to replace those in the softeners) are also available. | Resin can be effectively regenerated, meaning no long term build-up. Regenerant stream should be suitable for disposal (sufficient dilution possible). | From £500, plus resin cost. Low annual running cost (£20?) depending on water usage and type of system. | Well proven and effective system, although no tests done in UK. As such, a testing regime may be of benefit. The type of resin used is very important. Note: Not used as a softener. |
Ion-exchange Point-of-entry | >95% (dependent on resin) | As above. | As above. | Similar to above; higher running cost. | As above. Treating far more water than required. |
8. Quality assurance of point-of-use devices
Point-of-use and point-of-entry water treatment devices can be effective for treating the volumes of water needed for domestic and small commercial situations. Factors which should be considered when selecting a treatment device include:
- The efficacy of the treatment process
- The suitability of the treatment device for the water source being treated
- The monitoring and servicing requirements
- The potential effects of water contact materials and treatment chemicals on water quality
Most of the above points can be addressed at the design stage by looking at the device manufacturer’s or supplier’s published data. The data they supply should have undergone third party independent validation. In some case additional verification of the effectiveness of the final installation may be necessary depending on the risks involved or if the installed treatment option does not perform in line with design expectations.
8.1 Validation
Point-of-use or point-of-entry water treatment devices must have undergone validation to ensure that the claims of the manufacturer or suppler are valid. This is normally carried out against published test methods (for example a national standard).
8.1.1 Validation bodies
Validation should be carried out by an independent accredited third party organisation. This should be either a test laboratory or a product certifier. Test laboratories should be accredited to ISO 17025 and certification organisation to EN 45011 or ISO/IEC 17065 with the appropriate tests or product groups within their schedule of accreditation. The national accreditation body for test laboratories and certification organisation in the UK is UKAS (www.ukas.com); test laboratories and certification organisation in other countries are recognised in the UK by ILAC agreements (www.ilac.org).
8.1.2 Standards
The following standards, published by NSF/ANSI, are appropriate for point-of-use and point-of-entry water treatment devices. The standards contain several different contaminant removal tests and the installer or user should ensure that the claims validated are appropriate for the water to be treated:
NSF/ANSI 44 | Cation Exchange Water Softeners |
NSF/ANSI 53 | Drinking Water Treatment Units – Health Effects |
NSF/ANSI 55 | Ultraviolet Microbiological Water Treatment Systems |
NSF/ANSI 58 | Reverse Osmosis Drinking Water Treatment Systems |
NSF/ANSI 62 | Drinking Water Distillation Systems |
Products verified as conforming to the ANSI/NSF standards by third party certification bodies are available from a number of certification organisations.
Note 1: The NSF/ANSI standards do not address the effects of materials on water quality requirements of regulation 5 of The Regulations.
Note 2: Since 2000 standards relating to the performance, safety and testing of point of use devices have been published by CEN TC 164 as ENs. These standards are not suitable for the treatment of water for private supplies as they assume the incoming water meets the requirements of the Drinking Water Directive.
8.2 Installation and Verification
Devices will have been validated in accordance with the supplier’s installation, set up and operating instructions and these must be followed (including maintenance requirements) to ensure correct operation.
In certain circumstances it may be appropriate to verify the performance of the treatment device as part of the final installation. This can normally be carried out by comparison of appropriate samples of the water upstream and downstream of the treatment device once installed. Challenge dosing of the incoming water should not be carried out unless appropriate safeguards can be put in place to protect users during and after the challenge test is completed and the used challenge water can be disposed of safely and legally.
9. Treated water storage
A water supply system should include some form of treated water storage to provide a reserve of drinking water to cater for fluctuations in demand and in the event of planned maintenance or problems with the source or treatment. Storage may take the form of a small covered reservoir, providing sufficient head to supply more than one property, or may be a suitably positioned storage tank (e.g. in the roof space of the property), from which water flows under gravity to the taps. The tank or reservoir should hold a volume sufficient to accommodate the peak demand and the maximum period of interruption of supply, but must not be so large that water is allowed to remain static for lengthy periods because allowing the water to stagnate and develop aesthetic issues. The storage tank and other parts of the water supply system may be contaminated during construction and should therefore be disinfected before use. This is achieved by filling the system with a 20mg/l solution of chlorine and leaving it to stand for several hours, preferably overnight. The chlorine solution should then be drained off and the system rinsed thoroughly using treated water.
All storage tanks must be insulated to guard against freezing during the winter. Insulation will also prevent the water from excessive warming during the summer months, which could encourage both aesthetic and microbiological issues. The tank must be fitted with a robust lockable, and well fitting (but not airtight) lid to exclude light and pollutants. It is especially important to prevent the ingress of insects and animals and all openings must be protected using a fine mesh screen.
The storage tank must be inspected regularly; at least annually and preferably every six months. If necessary, any accumulated silt can be flushed or siphoned out and the system disinfected as described above.
10. Distribution systems
The system of pipes, channels and vessels that store and convey water following treatment (where present) to consumers’ taps is known as a distribution system or distribution network. It comprises one or more pipes, often with one or more connected reservoirs and/or tanks, and chambers, and are constructed from a variety of materials and water fittings (plumbing products). These systems may also include valves (of various types), hydrants, pumps, connection facilities and inspection points or other fittings for control and/or maintenance purposes. Even where effective treatment is used to secure a wholesome supply of drinking water, its quality at the point of consumption may not be wholesome if the integrity of the distribution system is compromised.
Unfortunately, the identification of risk on any distribution system can be difficult owing to the pipes and tanks etc. often being underground or hidden, which prevent or hamper inspection. Therefore the water quality impact of deficiencies in a system may not become evident until it manifests, either through monitoring (sampling and analysis), or more usually through objectionable taste, odour or appearance at the point of consumption.
The arrangement of distribution systems can be extremely variable, both in terms of their complexity and physical construction, and can range from simple single length pipe runs to larger more complex systems that are difficult to trace. In either case, even small networks can span distances from a few meters to several miles. Consequently,the number of potential or actual hazards present in any distribution system can be numerous, diverse and variable. In turn the risks that these present can and will have a direct bearing on water quality if the system is not adequately constructed, managed and/or maintained according to its layout and design, irrespective of its age, which in some cases might be significant.
Risks to water quality can be mitigated and controlled by an awareness of the hazards and sensible and simple management and maintenance of the system. For example, routine cleaning of storage facilities, having suitably sized robust and covered storage tanks and reservoirs, periodic flushing of the system through taps and hydrants and the use of approved water fittings (plumbing parts and substances). The inclusion of backflow protection devices such as check valves are also an essential component of any distribution system to prevent contaminants being sucked or pushed into the system through pressure differentials.
Further detail and advice can be gained from local authorities. The Water Fittings Regulations (1999) only apply to domestic plumbing fed from public supplies, however, they contains useful information on best practice for plumbing systems, especially regarding backflow protection and prevention of contamination of supplies. Further basic information and advice is shown in appendix X. Guidance also found – Private Water Supplies – Drinking Water Inspectorate (dwi.gov.uk)
11. Maintenance and training requirements
One of the most neglected aspects of small water supply systems is that of management and maintenance. Often the usual practice being to confront equipment failures and quality issues as they arise, leaving a risk of contaminated water until the problem is remedied. Maintenance should be proactive not reactive and involve a regular, preventive, maintenance programme, which enables early identification of problems. Equipment manufacturers’ maintenance instructions must be followed as a minimum requirement. Simple checks can be used to give forewarning of problems:
- daily (or more frequent) checks on the operation of disinfection equipment (e.g. check that the UV lamp is working and is on, measure chlorine residual to ensure it is within the recommended concentration range)
- investigate the causes of dirty or discoloured water as it could be due to system failure
- regular cleaning of filters in line with the manufacturer’s recommendations
- routine site inspections to check for signs of pollution of the water source
- structural inspections of the treatment plant, storage tanks and pipework
Maintenance should always be performed by those deemed competent (trained and/or experienced and familiar with the equipment), ideally by the supplier’s servicing contractors. At the time a water treatment plant is installed and commissioned, the supplier should provide training on routine operation and maintenance tasks. The training should cover:
- checking that treatment is operating correctly
- topping up chemicals as required
- routine maintenance of the equipment
- making simple repairs
- trouble shooting
This onsite training should be supported by appropriate documentation, such as an operation and maintenance manual. Evidence of competency is recommended and records of maintenance should be kept at all times.
Appendix A: Glossary of technical terms
The following is a list of working definitions of technical terms used in this manual. To aid comprehension, the definitions have been expressed in relatively simple terms, and as such are not necessarily scientifically exact.
Acid | A substance which in solution produces hydrogen ions (H+), the concentration of which is expressed in terms of pH units. |
Acidic | Having a pH value less than 7. |
Acidity | A measure of the capacity of a solution to neutralise alkali. |
Activated carbon | A family of carbonaceous substances manufactured by processes that develop adsorptive properties. Sometimes called “activated charcoal‟. |
Adsorption | The process by which one substance is taken onto the surface of another substance by physical and/or chemical forces. |
Aeration | The vigorous exposure of water to a stream of air bubbles. This process may increase pH by stripping carbon dioxide, remove odour-producing compounds and will increase the dissolved oxygen concentration to facilitate oxidation reactions (e.g. oxidation of iron). |
Algae | Microscopic planktonic plant forms which occur in unicellular, colonial or filamentous forms. Substantial growths („blooms‟) may be observed in nutrient-rich waters during the summer. |
Alkali | Any highly basic substance, for example, hydroxides and carbonates of alkali metals such as sodium and potassium. See base. |
Alkaline | Having a pH value greater than 7. |
Alkalinity | A measure of the capacity of a solution to neutralise acid. Note that there is a distinction between “alkaline‟ and „alkalinity‟ – e.g. a water of acid pH (i.e. not alkaline) may contain amounts of carbonate/bicarbonate which result in a degree of alkalinity. |
Anoxic | Water depleted of dissolved oxygen. |
Aquifer | A porous rock which holds and transmits water. |
Bacteria | Unicellular micro-organisms that typically reproduce by cell division. Many bacteria are disease-producing. |
Baffle | A slat, plate or plank used in a tank to alter the flow of water in order to avoid short-circuiting, e.g. in a chlorine contact tank. NB. in other contexts, baffles can be used to promote mixing. |
Base | Any chemical species capable of reacting with hydrogen ions. See alkali. |
Base exchange | A softening process using cation exchange resin to replace calcium and magnesium ions in the water with sodium ions. |
Breakpoint chlorination | A method of chlorination in which a sufficient dose of chlorine is added to rapidly oxidise all of the ammonia in the water and leave a free chlorine residual. |
Buffering capacity | The capacity of a water to resist pH change. This is closely related to alkalinity. |
Chloramines | Compounds formed from the reaction of chlorine with ammonia, successively replacing hydrogen atoms with chlorine atoms to produce monochloramine and dichloramine (and nitrogen trichloride if the pH is low and the chlorine:ammonia ratio is very high). Chloramines are less efficient disinfectants than chlorine itself but are more persistent. |
Chlorination | A process for disinfection of water using chlorine, sodium hypochlorite or calcium hypochlorite. |
Chlorine | A gas (Cl2) widely used in disinfection and oxidation processes. |
Coagulation | A process for turbidity and colour removal in which a coagulant (typically aluminium sulphate or ferric sulphate) is added to the water, causing colloidal turbidity-causing material and dissolved coloured substances to agglomerate into a precipitate („floc‟) which is subsequently removed e.g. by sedimentation and/or filtration. |
Coliform bacteria | A group of bacteria found in vast numbers in human excreta. Their presence in water is an indication of potential faecal contamination; consequently they are used as indicator organisms. See Escherichia coli. |
Colloid | A state of matter consisting of very finely divided (less than 1 micrometer) particles suspended in a fluid, e.g. water. Although the particles are not dissolved they are too small to settle at any meaningful rate. |
Colour | The shade or tint imparted to water, typically by organic substances in solution. |
Combined available chlorine | The total concentration of chloramines and any organic nitrogen chlorine containing compounds. Combined chlorine is a less effective disinfectant than free chlorine. |
Contaminant | Any undesirable physical, chemical, or microbiological substance in water. |
Cryptosporidium | A protozoan parasite which causes acute diarrhoeal disease in humans. The organism can be transmitted by contaminated water; both surface and groundwaters may be contaminated by the cyst stage of Cryptosporidium, known as oocysts. |
Cyst | A capsule associated with a dormant stage of reproduction in micro-organisms. See oocyst. |
Denitrification | The reduction of nitrate to nitrogen gas. Biological denitrification is a process in which reduction is brought about by naturally occurring bacteria. |
Desalination | The removal of dissolved salts (mainly sodium chloride) from sea water or brackish water to render it potable. |
Disinfection | The removal, destruction or inactivation of pathogenic organisms in water. |
Escherichia coli | The most abundant coliform organism present in the human and animal gut. The presence of E. coli in a water sample indicates potentially dangerous contamination of human or animal origin – see also coliform bacteria and indicator organism. |
Filter | A unit for carrying out the process of filtration which consists of a combination of filter medium and a suitable holder for constraining and supporting the medium in the path of the water. |
Filtration | The passage of water containing particles through a filter to effect a separation of particles from the water. |
Flocculation | A term often used interchangeably with coagulation, it refers to the process by which particles are agglomerated to form flocs (see coagulation). |
Flotation | A process for separating flocs from water. A recycled side-stream of water saturated with air under pressure is injected into the water. Microscopic air bubbles form at the point of injection, attach to the flocs and cause them to float to the surface where the accumulated solids can be removed. |
Free available chlorine | The total concentration of free chlorine, hypochlorous acid and hypochlorite ion in water. |
Gravel filter | A coarse filter for rapid filtration, consisting of a bed of gravel graded from coarse at the inlet to fine at the outlet. |
Hardness | Water hardness is caused by dissolved salts such as those of calcium and magnesium. Hard waters are associated with scale formation and increased use of soap; conversely soft waters are often acidic and corrosive. See total, permanent and temporary hardness. |
Hazen units (°H) | An arbitrary unit for expressing the colour of water; it can be represented as the platinum-cobalt (Pt-Co) scale in which colour is expressed in mg/l Pt. |
Hypochlorite | The hypochlorite ion (OCl–) is one of the ionic species formed when chlorine is added to water. The term „hypochlorite‟ is also used to refer to sodium hypochlorite, another chemical used for chlorination. |
Hypochlorous acid | Hypochlorous acid (HOCl) is one of the species formed when chlorine is added to water. |
Indicator organism | It would be futile and impractical to screen water samples for all possible pathogenic organisms. Escherichia coli and other coliform bacteria are therefore monitored as indicator organisms; their presence in a water sample would be indicative of faecal contamination and the potential presence of pathogenic organisms in water. |
Ion | An atom or group of combined atoms which has lost (or gained) one or more electrons to become a positively charged cation (or negatively charged anion). |
Ion-exchange | A process in which ions of like charge are exchanged between the water phase and the solid resin phase. |
Ion-exchange resin | A synthetic organic ion-exchange material, used for example in ion-exchange softeners and nitrate removal units. Typical resins are in the form of small beads. |
Marginal (simple) chlorination | The usual chlorination technique employed for small supplies of high quality. It is simply the dosing of sufficient chlorine to produce a suitable free chlorine residual. |
Membrane | A semi-permeable barrier that allows passage of water but not dissolved substances. Commonly used membranes are made of cellulose acetate and polyamides. |
Microfiltration | A pressure-driven membrane process using a membrane with pore size in the range 0.05 to 2 µm to separate suspended and colloidal material. |
Microgram per litre (µg/l) | A unit of concentration used in reporting analytical results at trace levels. For practical purposes it is the same as ppb (parts per billion). Note: 1000 µg/l = 1 mg/l. |
Micrometre (µm) | A linear measure equal to one millionth of a metre; also called a micron (µ). |
Microstrainer | A type of filter for removing mineral and biological solids, consisting of a rotating drum fitted with fine mesh panels. Water washing is used to remove accumulated debris. |
Milligram per litre (mg/l) | A unit of concentration used in reporting analytical results. For practical purposes it is the same as ppm (parts per million). |
Molecule | The smallest particle into which a compound may be divided and still retain the essential composition and properties of that compound. Molecules are composed of the atoms of individual elements. |
Nanofiltration | A pressure-driven process using a membrane with properties intermediate between reverse osmosis and ultra-filtration membranes. Nanofiltration membranes allow monovalent ions (e.g. sodium) to pass but selectively remove divalent ions (e.g. calcium). |
Nephelometer | An instrument used to measure turbidity of water. A nephelometer measures the amount of light scattered by suspended particles. |
Nitrification | The oxidation of ammonia to nitrite and nitrate. Biological nitrification is a process in which the oxidation is brought about by naturally occurring bacteria. |
NTU | Nephelometric Turbidity Unit. An arbitrary unit for expressing turbidity measured using a nephelometer. Also commonly used, closely related and practically numerically equivalent are Formazin Turbidity Unit (FTU) and Jackson Turbidity Unit (JTU) |
Oocyst | The dormant encysted form of a protozoan parasite such as Cryptosporidium. The oocyst is the environmentally stable, infective, form of the parasite. |
Oxidation | A chemical reaction in which the oxygen content of a compound is increased or in which a compound or ion loses electrons, thereby increasing its positive valency state (e.g. oxidation of ferrous to ferric iron). |
Ozonation | A process used for oxidation and disinfection using ozone. |
Ozone | A gas (O3) used as an oxidant and disinfectant in water treatment. Ozone is generated at the point-of-use using an ozoniser that employs high voltage electrical discharge through air or oxygen to produce ozone at a concentration of a few percent in the feed gas. |
Pathogen | A disease causing organism. |
Peak demand | The maximum momentary load (maximum water flowrate) placed on a water supply system. Domestic peak demand can be as great as 3 times the average daily demand. |
Permanent hardness | Hardness which is not precipitated by boiling. It is due to the presence of the chlorides and sulphates of calcium and magnesium. |
pH value | A logarithmic measure of acid or alkaline nature. pH 7 is neutral, acid solutions have a pH less than 7, alkaline more than 7. Each successive pH unit represents a tenfold change in acid or alkaline nature. |
Plumbosolvency | The contamination of water by lead resulting from dissolution of corrosion products from lead pipes and lead-containing solders or fittings. |
Point-of-entry | A term referring to the treatment of the entire supply of water delivered to or entering buildings or properties. |
Point-of-use | A term referring to treatment of water for drinking at the pointof- use or immediately prior to the point of delivery, i.e. typically at the kitchen tap, using for example a plumbed-in unit or system. |
Pressure filter | A type of filter consisting of graded sand layers contained within a closed steel vessel, through which water is passed under pressure. |
Private water supply | Any water supply which is not provided by a statutory water undertaker. |
Protozoa | Animal or vegetable organisms that consist of a single cell and which reproduce by fission. Most are microscopic, aquatic, and some are parasites. |
Rapid sand filter | Otherwise known as a rapid gravity filter, this consists of a bed of sand or other filtration medium contained in a concrete or steel vessel, through which water passes downwards by gravity. |
Reverse osmosis | A pressure-driven membrane process using a membrane with pore size below 0.002 µm to remove dissolved salts and organic compounds. |
Sedimentation | A process for removing suspended solids by passing water slowly and evenly through a lagoon, channel or tank to allow sediment to settle. Many different types of sedimentation system exist. Accumulated solids are removed periodically by appropriate mechanical or manual means. |
Slow sand filter | This consists of a layer of fine sand supported by a layer of gravel with a system of underdrains. Water is passed downwards at a low flowrate and solids accumulate at the sand surface, forming a biologically-active layer. Impurities are removed both by physical straining and biological action. |
Softening | Reduction of hardness (calcium and magnesium) by precipitation (lime or lime-soda softening) or ion-exchange (base exchange softening). Water conditioners which dose polyphosphates modify the hardness compounds, preventing them from precipitating and forming scale, but do not actually soften the water in the sense of removing calcium and magnesium. |
Superchlorination/ dechlorination | A chlorination process involving the addition of a large dose of chlorine to effect rapid disinfection and chemical reaction. This is followed by a dechlorination stage using e.g. sulphur dioxide or sodium bisulphite to reduce the excess free chlorine residual. |
Temporary hardness | Hardness which is precipitated by boiling. It is due to the presence of calcium and magnesium bicarbonate. |
Total hardness | A measure of the total amount of calcium and magnesium in solution. Total hardness is equal to the sum of permanent and temporary hardness. |
Trihalomethanes | A group of organic chemicals, including chloroform, which are formed in low concentrations during chlorination of waters containing natural organic compounds, such as the coloured humic and fulvic acids. |
Turbidity | An optical property of water resulting from the scattering of light by suspended and colloidal particles. Turbidity is determined using a nephelometer and the results give a measure of the amount of particulate matter present. |
Ultraviolet (UV) irradiation | A disinfection process using a mercury discharge lamp to irradiate the water, contained in a suitable chamber, with UVC radiation in order to inactivate micro-organisms. |
Virus | A sub-microscopic infective agent which may be a living organism or a complex protein capable of living in cells. Viruses cause a number of diseases in animals and plants. |
Appendix B: Distribution System Hazards and Mitigation
HAZARD | ISSUE | RISK | MITIGATION MEASURE |
---|---|---|---|
Long pipe lengths | Poor turnover: Water remains dormant for long periods, allowing it to stagnate. Elevated metals concentrations: Where pipes are metal, long exposure of water to the pipes may result in dissolution of metals into the water | Objectionable taste odour or appearance bacterial growth. Exceedance of metals standards (e.g. lead, copper, nickel). | Regular flushing of twice the capacity of the pipe. Reduce pipe run when possible. Ensure the system is not unnecessarily convoluted or long. |
Age, condition and material of pipes. | Potential for pipe material or component substances to dissolve (e.g. lead) or break off into the water (e.g. iron) | Cloudy water, water with particles, and possible objectionable taste and odour. Potential health effects depending on materials. | Replace or improve the pipes. Flushing in the short term may help, but it could make it potentially worse. |
Inappropriate location of pipes, vessels and chambers | Pipes and storage tanks/reservoirs located by waste facilities (latrines, toilets etc) or storage of contaminants such as oil could inadvertently leak into the supply | Contaminants may enter the supply leading to illness and/or taste, odour and appearance issues | Relocate pipes/vessels to a less hazardous location. Ensure tanks, chambers and tanks are in robust condition with protective measures in place, such as bunding around oil storage and ensure pipes are lined or are run in a protective sleeve to prevent chemicals migrating through plastic. |
Age, condition and material of storage vessels (tanks, reservoir, chambers) | Breakdown of materials into the water, potentially hazardous chemicals. Possible ingress points | Cloudy water, water with particles, objectionable taste and odour. Risk of decaying animals where they have gained access via holes, cracks etc. | Upgrade or replace the vessel. |
Contamination via ingress points | Inappropriate covers on inspection chambers or storage reservoirs or tanks, including loft tanks, will allow ingress of animals, including vermin and insects or encourage algal or bacterial growth | Ingress of land run off (pesticides, industrial pollutants), farm slurry or animal faeces from wildlife or grazing livestock | Inspection chambers, reservoir and tank roofs must have robust stock proof fencing. Ensure vents have a gauze covering, ensure chamber covers are adequately sealed and raised above the ground where possible. |
Low or irregular usage/little throughput of water | Low usage can encourage microbial growth or slime on the pipe walls called biofilms | Taste, odour and appearance problems. | Ensure tanks and reservoirs are of a suitable size to ensure regular turnover, ensure the system is not unnecessarily convoluted or long |
“Dead spots” in the system (areas of low or no flow such as redundant pipework or dead spots due to poor mixing). | As above. Water remains dormant for long periods, allowing it to stagnate, and this may get pulled back into the main flow of water. | Taste, odour and appearance problems | Remove dead legs and dead spots where possible. |
Fittings on the system that are unapproved, in poor condition or inappropriate | Unapproved materials from fittings products or treatment chemicals can impart chemicals to the water or encourage microbial growth in the system. | Taste, odour and appearance problems. Potential health issues depending on the circumstances and product. | Products should be replaced with appropriate approved materials and products. See DWI website for further details. (Transitional List of Approved Products) |
Back flow risks | Contaminants get pushed or sucked into the system due to pressure changes due to absence of backflow prevention devices. Possible contamination of the public supply. | Taste, odour and appearance problems. Potential health issues depending on the circumstances and contaminants. | Ensure appropriate protection devices are installed. Consult local water company. |
Uncontrolled operator access | Uncontrolled use of valve or hydrant operation | Inadvertent stirring up of sediment in the system leading to taste, odour or appearance issues. Connection of apparatus with inadequate backflow protection. | Restrict access to use of valves and hydrants. Document a procedure for their operation by approved personnel. |
Appendix C: Checklist for selection of treatment installers and verification of treatment solutions
What type of treatment do I need?
There are a number of treatment options available to choose from, the comparison table below shows the basic treatment capabilities of the most popular options.
Type of Treatment | Description | Contaminants treated | Uses | Advantages | Disadvantages | Installation cost | Ongoing maintenance cost (per yr) |
---|---|---|---|---|---|---|---|
Particulate Filters. | Filters of pleated papers, woven cartridges or ceramic candles. | Particulate matter (colour, turbidity) | To reduce discolouration and prepare water for further treatment | Simple, easy to use, no chemicals involved | Prone to blockage, can contaminate water with bacteria as bacteria can grow within the filters. | £ | £ Replacement of filter cartridges |
Activated carbon. | Utilisation of activated carbon to adsorb chemicals. | Colour, taste, odour, pesticides THMs. Will act as a physical filter to remove particles | Effective against target contaminants | Small, easy to use, no chemicals involved | Taste and odour from bacteria growth on the filter if not changed frequently enough. | £ | £ Replacement of filter cartridges |
Reverse osmosis units. | Physical filter the reverse osmosis process. | Usually used for chemicals which are difficult to remove with other treatments systems. Can be different pore size. | Usually used at point of use and blended with non-filtered water | Effective | Lots of waste water produced. Reduces minerals in the water to unacceptable levels. Can reduce pH to a level which corrodes pipework. | ££ | ££ Chemical cleaning of membranes |
Ion exchange units. | Swaps target contaminants on a media for anions or cations. Typically used in domestic water softeners. | Normally hardness and nitrate but different resins can be supplied for specific contaminants. | Typically used for hardness or nitrate removal | Excellent system for removal of nitrates. | Requires periodic backwash to regenerate resin. Requires replenishment of the salts periodically | ££ | £ Replacement brine solution |
Chlorine disinfection | Killing of micro-organisms through timed exposure to a chlorine dose | Bacteria and other microorganisms | Good for large supplies or supplies with a long network where the presence of a disinfectant residual is beneficial | Well-understood technology | Requires storage and use of chemicals, pH might need to be adjusted, requires contact time with the chlorine. Consumers may notice a taste. May require a pre filtration stage. | ££ | £ |
Ultraviolet disinfection (UV). | The passing of the UV light through the water to kill micro-organisms. | Bacteria and other microorganisms | Widely used. Can be particularly effective at inactivating Cryptosporidium. | Effective for the killing of microorganisms. | Requires regular cleaning and bulb replacement, and an electricity supply. May require a pre filtration stage as ineffective if water is turbid or highly coloured. | ££ | £ Replacement bulbs |
Iron and/or manganese removal | Oxidation of iron/manganese in water followed by filtration to remove it | Iron and/or manganese | Removal of iron/manganese | Often supplied as a single proprietary unit. Uses air for oxidation | Requires periodic backwash (although this may be automated) | ? Depends on size of unit | ? Depends on size of unit |
Cost approximate guide:
£ = <£100
££ = £100 – £1000
£££ – >£1000
When choosing a method of treatment there are a number of questions you should ask an installer to help you verify the suitability of the system for your needs and the capability of the installation company.
Checklist of questions you might like to ask an installer and responses you might expect from a competent person:
Questions: | Filtration | UV disinfection | Chlorine disinfection | Ion exchange treatment | Reverse osmosis | Iron and manganese removal | GAC |
---|---|---|---|---|---|---|---|
Do you have experience of this type of installation? | Yes | Yes | Yes | Yes | Yes | Yes | Yes |
What information would you need to know (or did you use) about raw water quality prior to selecting a treatment system? | Raw water turbidity; normal conditions and worst case. Analytical results for basic micro and chemical suite | Raw water turbidity; normal conditions and worst case. Analytical results for basic micro and chemical suite | Raw water turbidity; normal conditions and worst case. Analytical results for basic micro and chemical suite | Which chemical is to be removed? What are the levels of the chemical in the raw water in comparison to the standard? Is the contaminant likely to increase or decrease in the future? Is the contaminant seasonal? | Which chemical is to be removed? What are the levels of the chemical in the raw water in comparison to the standard? Is the contaminant likely to increase or decrease in the future? Is the contaminant seasonal? | Which chemical is to be removed? What are the levels of the chemical in the raw water in comparison to the standard? Is the contaminant likely to increase or decrease in the future? | Which chemical is to be removed? What are the levels of the chemical in the raw water in comparison to the standard? Is the contaminant likely to increase or decrease in the future? Is the contaminant seasonal? |
What information would you need to know (or did you use) about flows and demand prior to selecting a treatment system? | Average demand and peak demand. Future changes to demand such as additional properties or change of use. | Average demand and peak demand. Future changes to demand such as additional properties or change of use. | Average demand and peak demand. Future changes to demand such as additional properties or change of use. | Average demand and peak demand. Future changes to demand such as additional properties or change of use. | Average demand and peak demand. Future changes to demand such as additional properties or change of use. | Average demand and peak demand. Future changes to demand such as additional properties or change of use. | |
What did the risk assessment identify as potential contaminants? | |||||||
Do you only install Regulation 5 approved treatment systems and components? | Yes | Yes | Yes | Yes | Yes | Yes | Yes |
What pre-treatment might be required? | Filtration | Filtration | Filtration | Filtration | Oxidation of the iron or manganese using oxygen or chlorine | ||
How will I know if there’s a problem with the treatment system? | Discoloured water. Taste and odour problems if filters not changed frequently enough. Loss of flow/pressure if filter blocked | Alarms. Lamp replacement indicator (light or counter). Light if turned on. | Back-up chlorine dosing system. Alarms (low/high/no dose). Auto shutdown on turbidity or chlorine dose. | Alert if brine levels low. Water pressure may drop when media needs refreshing. | Loss of water pressure | Loss of pressure. Discolouration. | Loss of pressure. Discolouration. |
What ongoing maintenance will be or is required for the system? | Filter cartridges will need to be replaced periodically. | UV bulbs need to be replaced periodically. | Cleaning regime for quartz sleeve. Periodically more chemical stocks will be required. | Regeneration of the media is required periodically (check if manual or automatic). Media in the vessel may need to be replaced. Salt for regenerant solution will need replacement | Water remains clear and odour free | Cartridges or media will need to be replaced periodically. | Water remains clear and odour free. |
How will you confirm the system is effective? | Visual checks and turbidity measurement. Loss of pressure? | Raw and treated water samples to be taken to verify effective treatment.* | Raw and treated water samples to be taken to verify effective treatment.* Chlorine residual measurements | Raw and treated water samples to be taken to verify effective treatment.* | Raw and treated water samples to be taken to verify effective treatment.* | Raw and treated water samples to be taken to verify effective treatment.* | Raw and treated water samples to be taken to verify effective treatment.* |
Will a warranty be provided for the system? | Yes | Yes | Yes | Yes | Yes | Yes | Yes |
How much will the system cost to run? | Information | Information | Information | Information | Information | Information | Information |
Will you show me how to operate the system? | Yes | Yes | Yes | Yes | Yes | Yes | Yes |
Will you provide an operating manual for me to keep? | Yes | Yes | Yes | Yes | Yes | Yes | Yes |
Do you offer a maintenance contract? | Information | Information | Information | Information | Information | Information | Information |
How long will the system last? | Information | Information | Information | Information | Information | Information | Information |
How big will the system be? | Information | Information | Information | Information | Information | Information | Information |
What system is recommended? |
*if the sampling is carried out by the installer, ensure he is using a UKAS accredited laboratory for the analysis.
Further useful information:
Private Water Supply Regulations: Legislations relevant to PWS – Drinking Water Inspectorate (dwi.gov.uk)
Water Regulations Advisory Service: https://www.wras.co.uk/
http://www.privatewatersupplies.gov.uk/
Updated Report June 2015